1. Introduction
Depending on fossil fuels as our main energy source will cause in the mid-term an energy crisis and several environmental contamination problems. Hydrogen gas is one of the most promising alternatives to fossil fuels. The H2 gas is considered renewable, clean, and produces only water during its combustion. Among the different ways to produce hydrogen, biological production seems to be the most attractive alternative due to different products can be used as raw material, most of which are cost efficient and easy to find. Moreover, if the process is optimized, we can obtain byproducts with added value, for instance, organic acids and biopolymers [1]. Nevertheless, on the hydrogen production through dark fermentation, there are several factors that influence and limit the process, among which there are: pH value, temperature, C/N relation, carbon source, S/X relation, configuration of the reactor, fermentation route, the dominant microbial community, etc. [2,3].
The UASB reactor is the most widely used reactor in the world for treating domestic and industrial wastewaters. Ever since its creation by Gatze Lettinga in the 1980s, it has been an economically viable technology, easily used with promising results. The feasibility of UASB reactors for the production of hydrogen was initially studied by [4], who found values of 0.16 L. L-1.h-1 of Hydrogen Production Rate (HPR) operating at 2 h of HRT without presence of methane in the biogas, using an inoculum of mixed cultures and wastewater from the rice processing as substrate. [5] carried out similar experiments and observed that the granule formation was formed on the 173rd day of operation, and from that moment, the hydrogen production was stable for an 8-month period. [6] Studied the feasibility of producing hydrogen by treating cheese whey in UASB reactors and noted that the H2 production was of 112 mLH2. L-1.d-1 for an organic load of 20 g COD. L-1.d-1 and 2.5 g COD. VSS-1.d-1. These authors state that, although these values are low, the possibility of using UASB reactors fed with industrial wastewaters for the production of hydrogen was proven. [7] evaluated the production of hydrogen after the production of methane in UASB reactors by treating wastewaters from the cassava industry. They used as inoculum active sludge from an anaerobic lagoon previously treated with thermal shocks for inhibiting methanogenic organism and optimizing the production of hydrogen on its first stage. The results were promising not only because of the reactor used but also because was used real industrial wastewater. The maximum hydrogen production reached 37% with a volumetric organic load of 25 KgCOD.m-3 d-1. At a high load, the system collapsed and the hydrogen production was reduced mainly due to the accumulation of fatty organic acids.
Despite the already known advantages of UASB reactors, the practical application of these systems for producing hydrogen is still limited, mainly due to long initial times, the type of inoculum capable of minimising the biomass drag, proper handling of hydraulic detention times, etc. [8] mentioned that, by placing supporting structures at the suspension, the process could be accelerated; this is known as hybrid UASB. [9] used a hybrid UASB reactor to treat wastewaters with phenolic compounds. The reactor consisted of an acrylic cylinder at a bench scale in which approximately 54 rings were placed in a 30.48 cm in the middle of the reactor. Hence, a larger biomass concentration would be retained at shorter hydraulic retention time. The results showed that the anaerobic hybrid reactor tolerates 2.5 times more the phenolic organic load increase than the conventional UASB reactor. [10] also demonstrated that having an area with a filtering medium in suspended biomass anaerobic reactors improves the contact between the substrate and the sludge, minimizing the sludge loss and reducing the hydraulic retention time.
The Hydraulic Retention Time (HRT) is one of the operational variables that can be easily manipulated to optimize the hydrogen production. [11] compared the hydrogen production using glucose as a carbon source in a CSTR and UASB reactor with different HRT values (12-2 h). They discovered that there was more stability in UASB reactors and larger volumetric production values of H2, 19.05 mmolH2. L-1.h-1 for a 2 h of HRT. Research conducted by [12] treating wastewater with starch as a carbon source and activated carbon as support in an expanded bed anaerobic reactor concluded that the HRT with the highest volumetric production values was 4 h. These authors modified the HRT to a 24-4 h rate. Recently conducted research by [13] shows that unlike what was previously thought, at low HRTs in both conventional UASB reactors and packed bed reactors, the hydrogen production considerably decreases, being more noticeable at UASB reactors than at packed bed reactors.
In this sense, this research evaluated the effects of three different HRT on H2 production at conventional and hybrid UASB reactors, using on the top of the reactor a layer of polyethylene rings with foam of polyurethane in its center. This work could contribute to practical knowledge for the hydrogen production at UASB reactors.
2. Material and methods
2.1. Experimental design
Two Upflow Anaerobic Sludge Blanket Reactors (UASB) were used, a hybrid one (R1) and a conventional one (R2) with an internal diameter of 74 mm and a useful volume of 2.94 L and 3.0 L, respectively (Fig. 1). The Setting of the R1 reactor is characterized by the addition of a suspended bed of 100 mm in height composed of high-density polyethylene rings (Ø =19 mm and 10 mm in height) that contains a polyurethane foam core, with the purpose of avoiding sudden biomass washing and improving the biogas production stability [14,15].The specific surface area of each ring is 950 m2 .m-3.
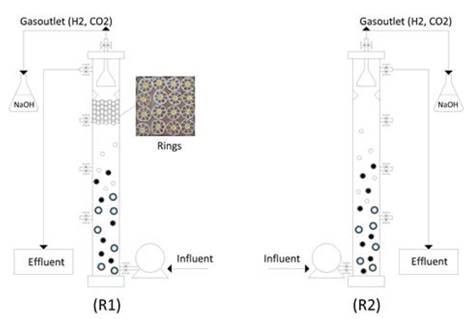
Source: The authors.
Figure 1 Schematic diagram of the UASB hybrid (R1) and conventional (R2) reactors.
The reactors operated with a constant flow for 134 and 104 days, respectively. The operation time for R1 was 12 h for 39 days and the second stage with an 8h for 48 days; and the third stage with a 4 h for 45 days. On the other hand, the R2 reactor was operated with a 12 h, 8 h and 4 h HRT for 45, 40 and 12 days, respectively. The reactors were placed in a thermocontolled chamber at a constant temperature of 35 ºC
The reactors were fed with synthetic wastewater using the sucrose as a carbon source. Additionally, an inorganic nutritional source used by [1] was added that contains CH4N2O (20 mg.L-1), NiSO4 6H2O (0.5 mg. L-1), FeSO4 7H2O (2.5 mg. L-1), FeCl3 6H2O (0.25 mg. L-1), CaCl2 2H2O (2.06 mg.L-1), CoCl2 2H2O (0.04 mg. L-1), SeO2 (0.036 mg. L-1), KH2PO4 (1.3 mg. L-1), KHPO4 (5.36 mg. L-1) and Na2HPO4 2H2O (2.76 mg. L-1). The volumetric organic load for the three operation stages was kept at 11.26 Kg COD m-3.d-1, which improve a stable hydrogen production at fixed biomass reactors using recycled pneumatic as support [16] (Mendez et al. 2017). The initial pH of the synthetic wastewater was adjusted at 5.5, adding HCL (10 M).
For both reactors, the inoculum was obtained using the natural fermentation mechanism. Some authors like [17-19] demonstrated that this fermentation that is caused by the combination between the microorganism present in the atmosphere and in the tap water is beneficial to maintain the acidogenic conditions.
2.2. Analytical methods
Percentage concentrations of H2, CO2 and CH4 were simultaneously measured using the gas chromatography equipment Agilent 7890A GC with a thermal conductivity detector (TCD) and a capillary column Carboxen 1010 plot, with a length of 30 m, 0.32 mm internal diameter and 25 µm of the internal stationary layer. The carrier gas was Argon and the injection volume was of 0.6 mL. The average values were the result of three measurements per sample.
The physical-chemical parameters of the influent and effluent samples were monitored three times per week. The organic content (COD), the total volatile acid amount (TVA), volatile suspended solids (VSS), and pH were assessed following the standard methods guideline [20]. The concentration of total carbohydrates on the influent and effluent of the reactor were analyzed using the phenol method [21].
2.3. Statistical analysis
The OriginPro (Version 8.0) software was used to conduct the statistical analysis of variance (ANOVA) of only one path to determine the statistically significant differences (p<0.05) between the measured and calculated parameter for each reactor at the different HRT. In addition, the Tukey test was conducted to do multiple comparisons between the parameter averages studied with 95% reliability. For each parameter, a statistical description was applied.
3. Results
From Figs. 2 and 3, it is evident that the suspended bed in the R1 reactor improves the system performance regarding stability in the hydrogen production. Not only the production is stable throughout the entire operation process, but the percentage value of the hydrogen gas also remains over 50%. This is not the case of the total volatile acid behavior, which despite a percentage production over 90%, there is no visible difference related to the type of reactor, indicating that its production is probably affected by the biomass inside the biological reactors. These results are confirmed with the ANOVA test, p=0.8203 for R1 and p=0.2194 for R2, and it shows that there was no significant difference on the TVA production for both reactors at the different studied HRT values. These observations contradict the stated by authors such as [22] Kim et al., (2013) who, from several experiments varying the HRT from 0.5 to 2.5 days determined that this parameter has a direct influence on the TVA production.
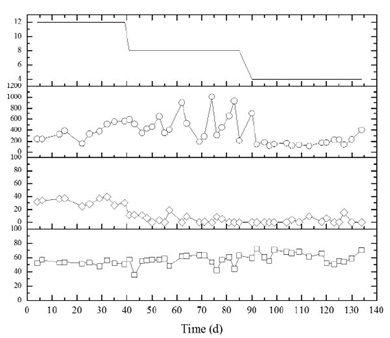
Source: The authors.
Figure 2 Performance of UASB Hybrid reactor (R1). ( ̶ ○ ̶ , Total volatile acids in the effluent as mgHAc/L, ̶ ◊ ̶ , production of CO2 in %, ̶ □ ̶ , production of H2 in %, - Hydraulic retention time).
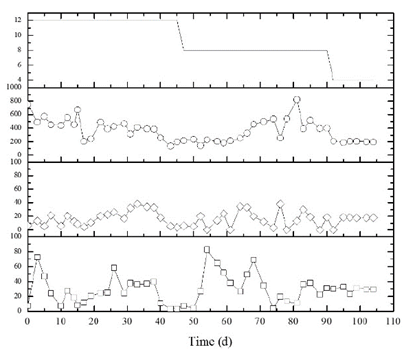
Source: The authors.
Figure 3 Performance of UASB Conventional reactor (R2). ( ̶ ○ ̶ , Total volatile acids in the effluent as mgHAc/L, ̶ ◊ ̶ , production of CO2 in %, ̶ □ ̶ , production of H2 in %,- Hydraulic retention time).
[23] on a deep revision about the anaerobic digestion emphasise that this technology is not only designed to treat wastewater with high organic loads and to produce methane, but it must also be planned to produce hydrogen separately or simultaneously with methane and other byproducts with high added value such as volatile fatty acids (VFA). It is important to mention that VFAs are considered to be probable precursors of the biopolymer production and other products such as biofuels, alcohols, aldehyde and ketones.
Although the purpose of this research was evaluating the influence of HRT on biohydrogen production in two different high rate anaerobic reactors, it was noted that the TVA results showed methanogenesis inhibition and very low pH values at the effluent, on average 3.4±0.2 and 3.3±0.2 for the R1 and R2 reactors respectively. This behavior was already mentioned by [24,25], who state that, when there is an accumulation of volatile organic acids in the system, there is also a pH reduction, causing destabilisation in the anaerobic process represented by inhibition in the methane production.
[6,26] show that there is both a minimum value of volatile acid where the inhibition of methanogenesis is produced and a maximum value where hydrogen-producing organisms are inhibited at 400 mgHAc. L-1 for the first and 10,000 mgHAc.L-1 for the second. On this research in particular, the minimum value for R1 was 101.95 mgHAc. L-1 (113th day of operation) and the maximum value was 1008.48 mgHAc. L-1 (74th day of operation). For R2, the values were 138.67 mgHAc. L-1 (43st day of operation) and 822.42 mgHAc. L-1 (81st day of operation). With these results, we can observe that the minimum values in which there is inhibition of methanogenesis are lower than the ones found in the literature.
At reactor R1, in which the hydrogen production was more stable, there was no accumulation of organic acids that would increase toxicity at hydrogen producers. A possible explanation to this observation can be that the pH value in the system was always kept at 3.5 units, which creates an extremely acid environment that permits that there are not associated this contributes to a reduction of toxicity amongst microorganisms.
On the other hand, [27] state that the organic loading rate (OLR) does have an influence on the variation and accumulation of organic volatile acids, unlike other parameters. Amongst the results presented by these authors, it is important to highlight the proportional increase in the total volatile acid concentration when they vary the organic load at molasses synthetic wastewater. The TVAs increased their concentration from 2.000 mg. L-1 to 7.000 mg.L-1 with values from 5 to 12 Kg COD. m-3.L-1, respectively. Furthermore, it is important to highlight that butyric acid shows the highest percentage at the total value.
Particularly, the organic loading rate (OLR) value was taken from previous results in reactors similar to ours, whose purpose was finding the load that would permit a good stability when producing hydrogen. These results can be consulted at Show and Tay, (1999) who found that, with load values above 11.26 Kg COD. m-3.d-1, not only was the system unstable when producing hydrogen, but there was also a percentage reduction of total volatile acids from 90% to 60%. The latter one indicates that there is a limit in the organic load value that optimises the production of organic acids and that it also guarantees stable and concomitant hydrogen production.
The results from ANOVA and the TUKEY test for reliability levels of 95% showed that there was a significant difference on the hydrogen production value for the R1 reactor (p=0.0033) during the 12 and 4 h; and the 8 and 4 h. As for R2, (p =0.4333), the result was different due to its high instability during the process. The average hydrogen production at reactor R1 was 53±3, 55±8, and 62±7 % for 12.8 and 4h, respectively. While the hydrogen production value for R2 did not exceed the average value of 35±21 % presented at the 8 h, previous results indicated that the hydrogen production especially depends on the reactor setting and on the operation using low HRT values. The latter is similar to the stated by authors such as [29-30], who showed that the H2 content in biogas is lower when the HRT value increases. This behavior is also detailed by [31,12], who mentioned that high HRTs allow the consumption of H2 through methanogenesis and homoacetogenesis at high rate continuous stirred tank reactors (CSTR), packed bed reactors (PBR), and UASB. Moreover, authors such as [32] indicate that low HRT values affect the ability to hydrolyse substrate and, therefore, affect the possibility of reaching high H2 production rates.
It is evident that the R2 reactor setting is less appropriate for the production of hydrogen. [7,33] mention that a biomass loss significantly affects both H2 production and the process stability. For instance, authors such as [3,34], who used hybrid UASB reactors with carbon nanotubes and plastic pall rings, respective, obtained H2 production values of 50%, similar to our R1 reactor, using a 12h . Whereas [5] obtained a H2 production of 42% using a conventional UASB reactor with an 8h HRT.
According to [25], the H2 content in the produced gas increases when the organic load reaches an optimal value of 68 Kg .m-3.d-1. Then, from that value despite the load increase of up to 79 Kg .m-3.d-1, the hydrogen percentage decreases about 30% whereas the CO2 content in the gas notably increases up to 80%. On our research, a larger concentration of hydrogen gas than carbon dioxide was observed. R1 showed CO2 values close to 0% especially after the 40th day of operation, which was the end of the 12 h. In the R2 reactor, the behavior was different; not only was it oscillating like hydrogen gas, but also 40% values were reached (Figs. 2 and 3). [35] mentions that low H2 productions are caused by non-H2 producing bacteria that produce, instead, excessive amounts of CO2 from the sucrose conversion.
The latter validates the already explained regarding the optimisation of a H2 producing system in the sense that HRT variations do not have an influence on the system’s stability, which particularly depends on the organic load value, the reactor’s setting and the inoculation methodology that, as it was observed, have improved the reactor’s performance thus allowing it to appropriately maintain the acidogenesis stage.
From Table 1, there is a comparison amongst the different types of reactors and the optimal conditions observed for the production of hydrogen. These results were more remarkable regarding percentage productions, which were higher than the previously mentioned, thus contradicting the stated by authors such as [25,36], who claim that thermophilic bacteria are more efficient for this kind of process.
4. Conclusions
This research verifies that placing Biopack® rings with polyurethane foam in its core, in UASB-reactors, allows its use for producing hydrogen. The maximum reached gas percentage was 62±7%, obtained at 4 h of HRT, thus showing that the process is favored by low hydraulic retention time. Furthermore, it is important to highlight that the support media not only allowed minimizing the loss of biomass, but also contributes to the stability of the hydrogen production if compared with a conventional UASB reactor. Lastly, it was observed that the 90% increase of total volatile acids in both conventional and hybrid UASB reactors had no effect on the hydrogen production.