1 Introduction
The main purpose of structural design is to obtain the best structural configurations and its member dimensions to support the imposed loads [1].
The traditional dimensioning process for footings takes a long time and the designer generally finishes the process after several iterations. The dimensions are determined from a procedure of successive approximations [2].
The dimensioning of the footings contact surface area on soil is usually done by admitting a linear distribution for the stresses transmitted to the soil. This hypothesis is quite likely in highly consolidated rocky or sandy and clay soils, and not for the latter when both are normally consolidated; in such a case, a parabolic distribution of stresses may be closer to reality [1-3].
The main contributions (Foundation optimization techniques are very popular because they present the best option for both dimensioning and design) on the topic of optimal design of shallow foundations that have been investigated recently are: Wang and Kulhawy [4] developed the economic design optimization of foundations. Chagoyén et al. [5] presented a rectangular shallow foundations optimal design and the formulation. Wang [6] developed the reliability-based economic design optimization of spread foundation. Kalinli et al. [7] proposed two approaches to obtain the ultimate bearing capacity of the shallow foundations, the first by means the artificial neural networks and the second by the ant colony optimization. Khajehzadeh et al. [8] presented a modified particle swarm optimization for optimum design of spread footing and retaining wall. Khajehzadeh et al. [9] studied the optimal design for shallow foundations by means the gravitational search algorithm. Basudhar et al. [10] developed the optimal cost-analysis and design of circular footings. Rizwan et al. [11] proposed a cost optimization of combined footings using modified complex method of box. Al-Ansari [12] investigated the structural cost of optimized reinforced concrete isolated footing. Khajehzadeh et al. [13] studied the multi-objective optimization of foundation using global-local gravitational search algorithm. Hassaan [14] proposed an optimal design of machinery shallow foundations with sand soils. Nama et al. [15] presented a parameters optimization of geotechnical problem using different optimization algorithm. Camp and Assadollahi [16] developed the CO2 and cost optimization of reinforced concrete footings subjected to uniaxial uplift. Sahoo and Kumar [17] presented a study for strip and circular shallow foundations on the ultimate bearing capacity by means of the finite elements, limit analysis, and optimization. Rezaei et al. [18] studied the thin-walled shallow foundations bearing capacity: an experimental study by means of the artificial intelligence. Ukritchon and Keawsawasvong [19] proposed a practical method for the optimal design of continuous footing using ant-colony optimization. El-Sakhawy et al. [20] showed an experimental study for the optimization of foundation shapes on soft soil. López-Chavarría et al. [21] presented a mathematical model for dimensioning of square isolated footings using optimization techniques: general case. López-Chavarría et al. [22] investigated the optimal dimensioning for the corner combined footings. Luévanos-Rojas et al. [23] investigated the optimal design for rectangular isolated footings using the real soil pressure. Gandomi and Kashani [24] used the recent swarm intelligence techniques to estimate the construction minimum cost of the shallow foundation. Velázquez-Santillán et al. [25] investigated the numerical experimentation for the optimal design for reinforced concrete rectangular combined footings. Jelusic and Zlender [26] investigated an optimal design of pad footing based on multi-parametric mixed-integer non-linear programming optimization. Malapur et al. [27] investigated an optimization of reinforced concrete column and footings using genetic algorithm. Rawat and Mittal [28] presented an optimization of eccentrically loaded reinforced concrete isolated footings. Nigdeli et al. [29] studied the metaheuristic optimization of reinforced concrete footings. These documents show the models for specific cases such as isolated footings and combined footings.
The mathematical models have been recently studied to obtain the dimensions of the isolated footings of shape: rectangular, square and circular [30-32]. Also the mathematical models have been presented to obtain the dimensions of the combined footings of shape: rectangular, trapezoidal, of strap and T-shaped [33-36]. These documents show the equations to obtain the dimensions of the contact area of the footings on the soil, but it does not guarantee the minimum area of the footings.
Therefore, the review of the literature clearly shows that there is no close relationship with the topic of optimal dimensioning of the contact surface on the soil for rectangular footings from 1 to n columns aligned on a longitudinal axis that is addressed in this paper.
This paper shows a new mathematical model for rectangular footings (general case) to obtain the most economical contact area on the soil (optimal surface) supporting from 1 to n columns aligned on a longitudinal axis (X axis), and each column subject to an axial load and two orthogonal bending moments in the connection with the footing. The proposed model considers that the soil pressure varies linearly. Also, numerical problems are shown to obtain the optimal contact surface on the soil for the rectangular isolated footings, and rectangular combined footings that support two and three columns (without restrictions on their sides, with one side restricted and two restricted opposite sides).
2 Formulation of the general model
Fig. 1 shows a rectangular footing that supports n columns aligned on a longitudinal axis (X axis), and each column provides an axial load and two orthogonal bending moments.
Fig. 2 presents the pressure diagram below the rectangular footing, and also the soil pressure in each corner on the footing.
The general equation to obtain the pressure anywhere on the footing due to the soil can be determined by [30-36]:
where: σ is the soil pressure anywhere on the footing, S is the contact surface of the footing, P is the axial load applied at the center of gravity of the footing, M x is the moment around the X axis, My is the moment around the Y axis, x is the distance parallel to the X axis measured from the center of gravity to the point in study, y is the distance parallel to the Y axis measured from the center of gravity to the point in study, Iy is the moment of inertia around the Y axis, and I x is the moment of inertia around the X axis.
The geometric properties of the contact surface of the rectangular footing with the soil are:
where: L x is the distance in X direction of the footing, L y is the distance in Y direction of the footing.
Substituting the eq. (2)-(4) into eq. (1) to find the stresses in each corner of the rectangular footing, these are obtained as follows:
where: R is the sum of all the axial forces, M x T is the sum of all the bending moments around the X axis and M y T is the sum of all the bending moments around the Y axis. These are obtained from the following equations:
The pressure generated by the soil must be equal or greater to zero and less or equal to the soil available allowable load capacity σ p .
The conditions of the property limit are:
1. There are no restrictions in the sides of the footing:
2. One of the sides of the footing is restricted:
3. Two opposite sides of the footing is restricted:
2.1 Optimal surface
The objective function for the minimum surface S min is:
The constraint functions for the minimum surface are:
Unrestricted sides
where: La ≥ C 1/2. One side restricted
Two opposite sides restricted
Numerical problems
Three types of numerical problems are presented below: The first numerical example is for rectangular footings that support a column (rectangular isolated footings). The second numerical example is for rectangular footings that support two columns (rectangular combined footings). The third numerical example is for rectangular footings that support three columns (rectangular combined footings). The Tables present three cases for the dimensioning of the rectangular footings varying the soil available allowable load capacity of "op = 250, 200, 150 kN/m2", and four types varying the mechanical elements acting on the footing. For cases 2 and 3 the separation between the columns varies from 4 to 7 m. The dimensions of the columns in all cases are of 40x40 cm.
3.1 Example 1
Table 1 shows the axial load and two orthogonal bending moments that act on the footing, the optimal dimensions, and the stresses that are generated in each corner of the rectangular footings that support a column (rectangular isolated footings) (see appendix).
3.2 Example 2
Table 2 shows the axial load and two orthogonal bending moments due to the column 1 and the column 2 that act on the footing, the optimal dimensions, and the stresses that are generated in each corner of the rectangular footings that support two columns without restrictions in sides (rectangular combined footings) (see appendix).
Table 3 shows the axial load and two orthogonal bending moments due to the column 1 and the column 2 that act on the footing, the optimal dimensions, and the stresses that are generated in each corner of the rectangular footings that support two columns with a side restricted (rectangular combined footings) (see appendix).
Table 4 shows the axial load and two orthogonal bending moments due to the column 1 and the column 2 that act on the footing, the optimal dimensions, and the stresses that are generated in each corner of the rectangular footings that support two columns with two opposite sides restricted (rectangular combined footings) (see appendix).
3.3 Example 3
Table 5 shows the axial load and two orthogonal bending moments due to the column 1, column 2 and column 3 that act on the footing, the optimal dimensions, and the stresses that are generated in each corner of the rectangular footings that support three columns without restrictions in sides (rectangular combined footings) (see appendix).
Table 6 shows the axial load and two orthogonal bending moments due to the column 1, column 2 and column 3 that act on the footing, the optimal dimensions, and the stresses that are generated in each corner of the rectangular footings that support three columns with a side restricted (rectangular combined footings) (see appendix).
Table 7 shows the axial load and two orthogonal bending moments due to the column 1, column 2 and column 3 that act on the footing, the optimal dimensions, and the stresses that are generated in each corner of the rectangular footings that support three columns with two opposite sides restricted (rectangular combined footings) (see appendix).
4 Results
In Table 1 is observed the following results: The types 1 and 2 in all the cases, the optimal surface, the dimensions and the stresses in each corner of the footing are equal. For the types 3 and 4, when the soil available allowable load capacity decreases, the optimal surface and the dimensions increase. For the first two types in all cases, it is limited by the minimum stress that is zero. For the last two types in all cases, it is limited by the soil available allowable load capacity.
In Table 2 is showed the results for the rectangular combined footings that support two columns without restrictions in sides: The type 1 in all the cases, the optimal surface, the dimensions and the stresses in each corner of the footing are equal. For the types 2, 3 and 4, when the soil available allowable load capacity decreases, the optimal surface increases. For the first type in all cases and the second type of the case 1, it is limited by the minimum stress that is zero. For another types, it is limited by the soil available allowable load capacity.
In Table 3 is presented the results for the rectangular combined footings that support two columns with a side restricted: For all types in all cases are presented the same results for the rectangular combined footings that support two columns without restrictions in sides (see Table 1) with the exception of type 4 for the three cases that show different sides of the footing.
In Table 4 is observed the results for the rectangular combined footings that support two columns with two opposite sides restricted: The types 1, 2 and 3 in all the cases, the optimal surface, the dimensions and the stresses in each corner of the footing are equal. For the type 4, when the soil available allowable load capacity decreases, the optimal surface and the dimension Ly increase, and the dimension Lx does not change because it is restricted. For the first three types in all cases, it is limited by the minimum stress that is zero. For the last type in all cases, it is limited by the soil available allowable load capacity. Also the resulting moments around the Y axis have a value and these are negative, this means that the resultant forces do not coincide with the center of gravity of the footing.
In Table 5 is showed the results for the rectangular combined footings that support three columns without restrictions in sides: The type 1 in all the cases, the optimal surface, the dimensions and the stresses in each corner of the footing are equal. For the types 2, 3 and 4, when the soil available allowable load capacity decreases, the optimal surface increases. For the first type in all cases and the second type of the case 1, it is limited by the minimum stress that is zero. For another types, it is limited by the soil available allowable load capacity.
In Table 6 is presented the results for the rectangular combined footings that support three columns with a side restricted: For all types in all cases are presented the same results for the rectangular combined footings that support three columns without restrictions in sides (see Table 5) with the exception of type 4 for the case 1 that shows different sides of the footing.
In Table 7 is observed the results for the rectangular combined footings that support three columns with two opposite sides restricted: The types 1 and 2 in all the cases, the optimal surface, the dimensions and the stresses in each corner of the footing are equal. For the types 3 and 4, when the soil available allowable load capacity decreases, the optimal surface and the dimension Ly increase, and the dimension Lx does not change because it is restricted. For the first two types in all cases, it is limited by the minimum stress that is zero. For the last two types in all cases, it is limited by the soil available allowable load capacity. Also the resulting moments around the Y axis have a value and these are negative, this means that the resultant forces do not coincide with the center of gravity of the footing.
Fig. 3 shows the optimal surface of example 1 of the three cases of rectangular footings that support a column (rectangular isolated footings). It is clearly observed that when the moments decrease, the contact surface decreases in all cases.
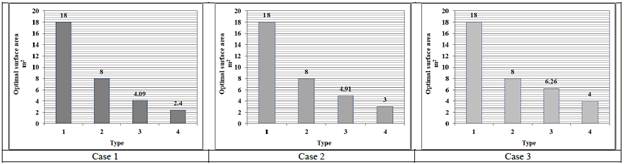
Source: Prepared by the authors.
Figure 3 Optimal surfaces for rectangular footings that support a column.
Fig. 4 shows the optimal surface of the example 2 for rectangular footings that support two columns (rectangular combined footings). The comparison of the optimal surface for sides without restrictions, for a restricted side and for two opposite sides restricted of the same type in each case is observed that the rectangular footings with two opposite sides restricted have greater contact surface with respect to the rectangular footings with a restricted side and sides without restrictions. For the three cases is observed that when the moments decrease, the contact surface decreases in all cases.
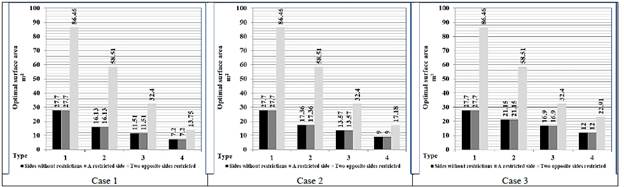
Source: Prepared by the authors.
Figure 4 Optimal surfaces for rectangular footings that support two columns.
Fig. 5 shows the optimal surfaces of the example 3 for rectangular footings that support three columns (rectangular combined footings). The comparison of the optimal surface area for sides without restrictions, for a restricted side and for two opposite sides restricted of the same type in each case is observed that the rectangular footings with two opposite sides restricted have greater contact surface with respect to the rectangular footings with a restricted side and sides without restrictions. For the three cases is observed that when the moments decrease, the contact surface decreases in all cases.
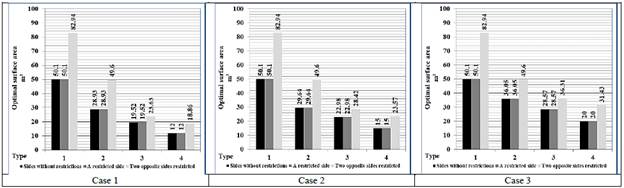
Source: Prepared by the authors.
Figure 5 Optimal surfaces for rectangular footings that support three columns.
The types 1 and 2 are same for the three cases of the three examples, because the soil pressure is governed by zero pressure, i.e., it does not reach the available permissible load capacity of the soil.
5 Conclusions
This paper shows a general model for rectangular footings to obtain the most economical contact area on the soil (optimal surface) that supports from 1 to n columns aligned on a longitudinal axis. The proposed model considers that the soil pressure varies linearly.
The models normally used to obtain the dimensions are: the dimensions are proposed and these are verified so that they are within the allowed limits, i.e., the pressure generated by the soil must be equal or greater to zero, and less or equal to the soil available allowable load capacity.
The traditional dimensioning process for footings takes a long time and the designer generally finishes the process after several iterations. The dimensions are determined from a procedure of successive approximations.
The main conclusions are:
When the moments around of the Y axis are zero, this means that the resultant forces match the center of gravity of the footing (see Tables 2, 3, 5 and 6).
When the moments around of the Y axis differ from zero, this means that the resultant forces do not match the center of gravity of the footing (see Tables 4 and 7).
The methodology shown in this document is more precise and converges more quickly with respect to the current model that is based on trial and error.
The traditional model for footings will not be practical compared with this model, because the traditional model for footings takes a long time and the dimensions are determined from a procedure of successive approximations.
The proposed model can be used for the dimensioning of rectangular isolated footings (see Table 1).
The proposed model can be used for the dimensioning of rectangular combined footings that support two or more columns aligned on a longitudinal axis (X axis) for sides without restrictions, a side restricted and two opposite sides restricted. For footings that support two columns (see Tables 2, 3 and 4). For footings that support three columns (see Tables 5, 6 and 7).
The model presented in this paper is effective tool in finding the optimal contact surface of the rectangular footing on soil that support one, two or more columns. This model is generated from of the loads and orthogonal bending moments that provide the columns aligned on a longitudinal axis (X axis).
The proposed expressions directly offer the dimensions of the contact surface of the rectangular footings on soil, also guaranteeing that the admissible stress in the soil will not be exceeded.
The investigations to consider in the future are: 1) Rectangular foundation slabs. 2) Foundations of type "L" of corners for two or more columns aligned on each axis (X and Y axes). 3) Foundations of type "U" for two corners.