Introduction
The physiological response of plants to water déficit is complex because a wide range of metabolic processes are affected (Lawlor & Tezara, 2009). Drought lead to a series of changes that produce lower CO2 assimilation rate (A). The decreased diffusion of CO2 to carboxylation sites due to stomatal closure has been proposed as an important factor in this process (Chaves et al., 2009). Other non-stomatal limitations also lead to a reduction in photosynthesis under water deficit (Tezara et al., 1999). During drought, there are also limitations on plant nutrient uptake from the soil and a consequent decline in nutrient concentrations due to lower transpiration rates (Gessler et al., 2016).
Cacao is a shade-tolerant species found in tropical regions under shade trees or without shade at altitudes between 0 and 500 masl and with an average annual precipitation above 1500 mm. Despite these precipitation levels, cacao is subjected to periods of drought that vary in duration and severity between regions (Ofori et al., 2014; Tezara et al., 2020). Long periods of drought can have substantial negative impacts on cacao tree growth, where younger trees are more sensitive than older ones (Moser et al., 2010; Lahive et al., 2018). Cacao responds to drought by closing stomata, causing a reduction in A and transpiration rat; E (Araque et al., 2012; Avila- Lovera et al., 2016). Drought also causes changes and limitations in nutrient uptake, which vary between cacao clones (Santos et al., 2014). However, the effects of irrigation or drought on cacao yield remain unclear (Carr & Loockwood, 2011).
Over the last 15 years, cacao plantations in Ecuador have experienced reduced levels of shade and this condition can rapidly affect plant metabolic processes during drought periods. However, the weather is mostly overcast during dry seasons (DS), leading to lower air evaporative demands and fewer high light hours, which determines a slower onset of water deficit. Ecuadorian clones have shown, on average, an increase in A and acclimation of some physiological and morphological traits with increasing photosynthetic photon flux density (Tezara et al., 2015; Jaimez et al., 2018; Suárez et al., 2018).
In Ecuador the frequency of high temperatures has increased in the 1951-2010 period. Furthermore, despite an increased frequency in extreme rainfall events in some regions of Ecuador, there has been a consistent increase in the number of consecutive days without rainfall (Donat et al., 2013). Physiological responses after 150 days of drought are unknown in cacao and will be important to know differences of tolerance to drought between clones to use in breeding programs. To address these concerns, we examined seasonal changes in water status, gas exchange, photochemical activity, leaf macronutrient concentrations and yield in 10 clones of Ecuadorian cacao in order to gain knowledge of the effects of drought on physiological traits and their relationship with the cacao yield. We expect a negative effect of drought on physiological performances and yield.
Materials and methods
Experimental design and climate conditions
Measurements were carried out in an established seven-year-old cacao plantation in Quevedo, Ecuador (1° 4’ 33’’ S, 79° 29’ 15’’ W) at an altitude of 110 masl. The plantation includes 30 clones, which were previously propagated by grafting onto seedlings of the 'IMC 67’ rootstock, obtained from open-pollinated seeds. The plantation was a randomized complete block design with two blocks, and each block consisted of 30 rows of 5 plants planted at 3 m, for a total of 10 plants per clone (see Jaimez et al., 2018). For this study, only eight Nacional clones still under evaluation to be released coded by the Cacao and Coffee Breeding Program of National Institute of Agricultural Research (INIAP) as: T1, T8, T12, T14, T17, T23, T24, and T28, were selected based on higher yield and disease tolerance to frosty pod rot (caused by Moniliophthora roreri). The clones come from crosses of CCN 51 with Nacional clones selected on characteristics of yield, organoleptic quality or tolerance to diseases (Table 1). Two clones (EET 103 and CCN 51) were also included. EET 103 is a commercial Nacional clone planted in various regions of the country and CCN 51 is a high yield Forastero clone.
Table 1 List of 10 clones of Ecuadorian clones evaluated and their genetic background (parental cross)
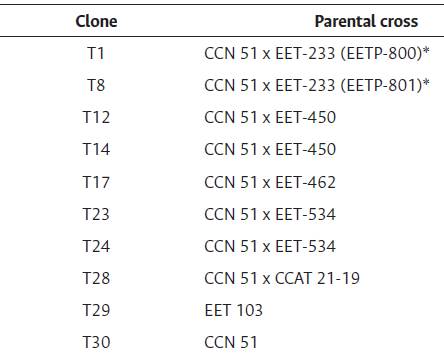
T1 and T8 have recently been released to farmers and are known as EETP- 800 and EETP-801.
During the plantation period (from August 2008 to July 2015), average air temperature and relative humidity were 24° C and 82 %, respectively. Maximum water vapour pressure deficit (VPD) fluctuated between 1.3 and 1.9 KPa from 13:30 h to 16:30 h. Mean annual precipitation was 2210 mm, characterized by two marked seasons: a rainy season (RS) from November to May, with a total rainfall average of 2124 mm; and a DS, from June to November, with 86 mm (National Institute of Meteorology and Hydrology, Pichilingue weather station). The soil was a volcanic andisol, characterized by 32 %, 54 %, and 14 % sand, silt, and clay, respectively and pH was 5.8. Cacao plants received a yearly fertilization of 200 g plant-1 of commercial formula NPK (10-30-10) and 100 g of urea, to guarantee well physiological and productive performance.
Soil water capacity and relative leaf water content
Evaluations took place under field conditions in the DS (at 130 and 133 days without precipitation; 21 and 23 October 2015) and RS (3 and 5 February 2016). Under laboratory conditions, the water retention capacity of the soil was determined at -33 (field capacity), -456 and -1500 KPa (wilting point). Soil water content (SWC), during physiological measurements was determined by the gravimetric method in three samples at a depth of 0-0.2 and 0.2- 0.4 m from the surface. Leaf relative water content (RWC) was determined from leaf discs (six discs per leaf, one leaf per plant, four leaf per clone; n = 4) for which the fresh mass (FM) was measured. Samples were then hydrated in distilled water for 5 h for measurement of turgid mass (TM) and then placed in an oven (65 °C) for 40 h to determine dry mass (DM). RWC was calculated as FM-DM/TM-DM.
Gas exchange
Measurements of instantaneous: CO2 assimilation rate (A), transpiration rate (E), stomatal conductance (gs), and water use efficiency (WUE) were performed in fully expanded leaves using a portable infrared gas analyser (CIRAS 2, PP Systems, Hitchin, UK) connected to an assimilation chamber (PP Systems) and a LED light unit. The WUE was calculated as A/E. Measurements were made at 1000 ± 4 μmol m-2 s-1 of photosynthetic photon flux density (PFD), an external CO2 concentration of 400 ± 10 μmol mol-1, leaf temperature of 28 ± 1°C and a Vapour pressure deficit (VPD) of 1.6 ± 0.3 KPa. These measurements were made on two days of each season on the third intact mature, fully expanded leaf belonging to a mature branch of six plants (n = 6) per clone (three per block) selected at random. Measurements were carried out between 10:00 h and 12:00 h to ensure high gs (Jaimez et al., 2018) and therefore the potential for the highest values of A to be achieved.
Photochemical variables
Chlorophyll fluorescence measurements were made using a portable fluorimeter (PAM 2100, Heinz Walz GmbH, Germany). Chlorophyll fluorescence was measured simultaneously on the same leaf where gas exchange was evaluated. Fluorescence parameters were evaluated using six plants (n = 6) per clone under the same PFD used during leaf gas exchange measurements, i.e. (1000 μmol m-2 s-1). Calculated photochemical variables were: relative quantum yield of photosystem II (ΦPSII) was calculated as ΦPSII = (F'm -Fs )/Fm , where F'm and Fs and steady state fluorescence in light-adapted leaves, respectively. Non-photochemical quenching (NPQ) was calculated as NPQ = Fv-(F'v/Fv), where F'v is the maximum variable fluorescence in any light adapted state and Fv is the maximum variable fluorescence when all non-photochemical process are minimum, i. e. dark-adapted leaves.
Leaf macronutrient determination
For each measurement period, three samples of mature leaves from three plants (n = 3) of each clone were harvested and dried at 70 o C for N, P, K, Ca and Mg determinations. Kjeldahl method was used for N, while for P, K, Ca, and Mg was carried out through the mineralization of the samples by wet digestion with nitric-perchloric mixture. Phosphorus content was quantified by colorimetry and the determination of K, Ca, and Mg was made by atomic absorption spectrophotometry. Results are expressed as percentages of dry matter.
Yield data
Yield was measured in ten cacao tree (n = 10) per each clone and cacao bean fresh weight per plant was registered during years 2012, 2013, and 2014. Total annual rainfall for years 2012, 2013, and 2014 were 3304, 2070, and 1592 mm, respectively.
Statistical analysis
All variables were analysed in a factorial design with 10 clones and two different soil moisture values (dry and rainy season) with six replicates. ANOVA was performed at a significance of p < 0.05. Differences between mean parameters among clones for each season were determined using Tukey test (p < 0.05) in SPSS (version 23, IBM USA). Relationships between content of different macronutrients were tested by means of simple regressions. All data are shown as mean ± standard error.
Results
Soil water content and relative leaf water content
Drought period started in mid-June during the measurement year. Under the dry period in October, soil moisture percentage was between 28 % and 32 % at 0-0.2 m and 0.2-0.4 m in depth, respectively; compared to a RS moisture percentage in February of 42 % and 40 % at 0-0.2 and 0.2-0.4 m, respectively (Table 2). Mean soil moisture percentage obtained at 0.033 and 1.5 MPa in the laboratory were 45.4 % and 21.6 %.
Table 2 Soil water moisture measured.

Soil water moisture measured at -33, -456, and -1500 KPa determined in laboratory conditions and soil water moisture in the field for dry and rainy seasons. Values represent three replications for laboratory determinations (n = 3) and six for measurements in the field (n = 6). All values are means ± standard error.
The interaction season x clone for RWC was not significant (p > 0.05), however mean RWC was significantly higher (74.9 ± 0.7 %) in RS than those in DS (68.9 ± 1.1 %). Clones T1, T17, T23, T28, EET 103, and CCN 51 showed significantly higher RWC (71.7-77.3 %) during RS (p < 0.05), while the remaining clones RWC did not change between seasons. The CCN 51 had the lowest RWC (61.5 %) during DS (Table 3).
Gas exchange
On average, drought caused a 33 % reduction in A, from 10.3 ± 0.9 to 6.9 ± 0.7 μmol m-2 s-1 (p < 0.05). The interaction clone x season for A was significant (p < 0.05). Clones T1, T8, T12, T14, T17, and T28 showed significantly higher A during RS, while clones T23, T24, EET 103, and CCN 51 did not change seasonally. During drought, T23 showed a significant higher A with respect to T12, T14, and T17 (Figure 1 A).
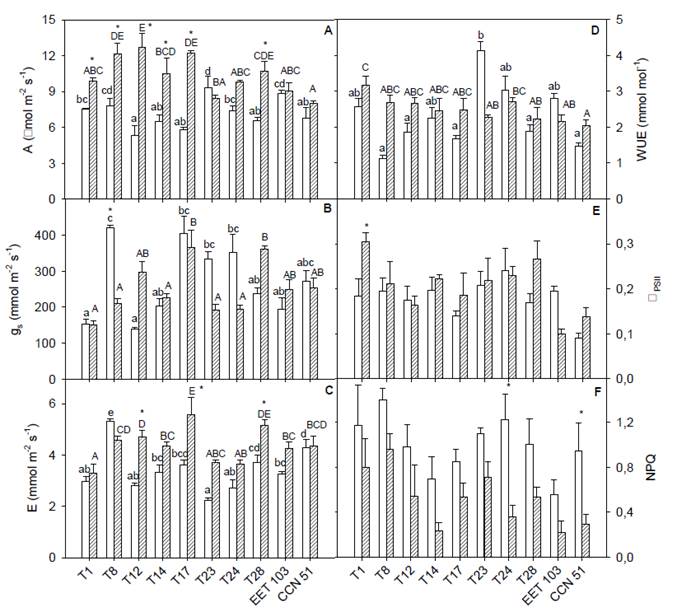
Figure 1 A, CO2 assimilation rate; B, stomatal conductance; C, transpiration rate; D, water use efficiency; E, relative quantum yieLd of PSII; F, non- photochemical quenching coefficient in Ecuadorian cacao clones measured during the dry (open bars), and the rainy (striped-bars) seasons. Different small letters represent significance between clones during DS; different capital letters represent significance between clones during RS. Asterisks (*) represent a significant difference between dry and rainy season conditions for the same clone.
The interaction clone x season for gs was significant (p < 0.05). The T8 showed a significant different gs between seasons, and this clone showed the highest gs during drought. T12 and T28 showed higher gs during RS (Figure 1 B). The interaction clone x season for E was significant (p < 0.05). During drought E mean showed a 22 % of reduction. With the exception of T8, all clones exhibited lower E during drought. Clones T12, T17, and T28 showed significant highest E during RS (Figure 1 C). The interaction clone x season was significant for WUE (2.3 ± 0.3 and 2.5 ± 0.1 mmol mol-1 during DS and RS, respectively). During RS, T1 showed higher WUE in relation to T23, T24, T28, EET 103, and CCN 51, whereas during drought, T23 exhibited a significant highest WUE (Figure 1 D). Low relationship was found between A and gs (r2 = 0.10, p < 0.05). Lower RWC values resulted in lower values of A (r2 = 0.30, p < 0.05, Figure 2).
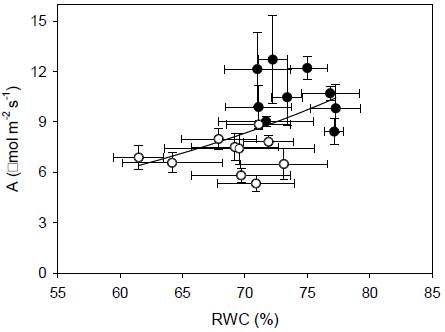
Figure 2 Relationships between CO2 assimilation rate and relative water content in ten Ecuadorian cacao clones, during dry (open circles) and rainy seasons (closed circles). The regression including both seasons all clones is represented by the solid line, ; r2 = 0.30.
Chlorophyll fluorescence
Non-significant differences were observed in ΦPSII between seasons (p < 0.05); whereas T1 showed trie highest for RS (Figure 1 E). For most clones, there was a significant increase for NPQ under drought (Figure 1 F); the mean NPQ was 0.87 ± 0.06 during drought which was significantly higher compared to 0.56 ± 0.04 in RS. A significant negative correlation was found between the NPQ and the RWC (r2 = 0.28, p < 0.05).
Leaf macronutrient content
Leaf macronutrient content was affected by drought and differences were found between clones. The interaction clone x season for N was significant (p < 0.05). The T17, T28, and ETT 103 clones showed a significant increase in N during drought. There were only significant differences in P between seasons (p < 0.05), where the mean P content value of RS was significantly higher than DS. Phosphorus content was similar in both seasons for all clone. Mean K content during drought was significantly higher than for RS (p < 0.05). Clones T8, T14, T17, T24, T28, and CCN 51 exhibited significantly higher K concentrations during drought (p < 0.05). Calcium content showed significant interaction clone x season (p < 0.05), T17 and T12 clones decreased during drought (p < 0.05). There was a significant linear relationship between Ca and E (r2 = 0.52, p < 0.05). Mean Mg content for RS was significantly higher than under drought (p < 0.05). T1 and CCN 51 exhibited significantly higher values of Mg content during RS than in drought. The rest of the clones showed similar Mg contents between seasons (Table 4). There was a significant linear relationship between leaf Mg content and RWC (r2 = 0.54, p < 0.05, Figure 3).
Table 4 Leaf macronutrients content (%) of Ecuadorian cacao clones for the rainy and the dry seasons Values are means of three samples ± standard error.
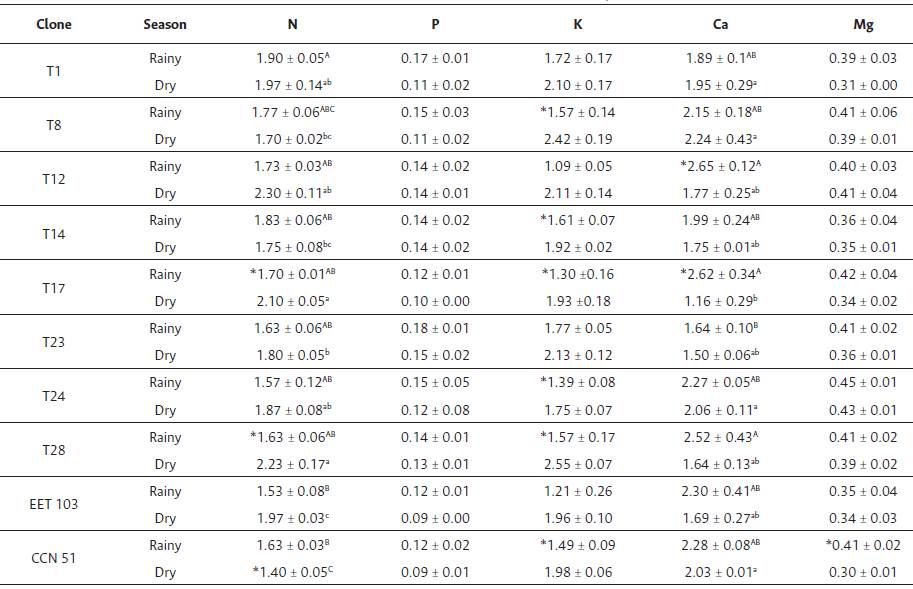
Capital and small letters represent significance between clones (p < 0.05). Asterisk (*) represents a significant difference between during the rainy and dry season, respectively within column dry and rainy seasons for the same clone according to Tukey's t-test (p < 0.05).
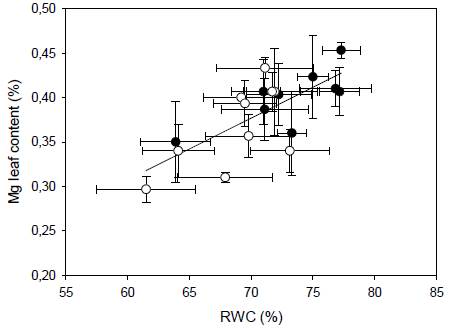
Figure 3 Relationships between leaf Mg content and relative leaf water content in ten Ecuadorian cacao clones during dry (open circles) and rainy seasons (closed circles). The regression including both seasons all clones is represented by the solid line, [Mg] = 0.0069 x RWC - 0.1093; r2 = 0.52.
Yield
Clones showed significant differences in fresh bean yield between seasons (p < 0.05). Yields of T1, T8, and CCN 51 were 33 %, 39 %, and 45 %, respectively, higher under drought; whereas in T17 and T24 yields were 48 % and 36 % higher during RS (Table 5). A low production was observed in clone T28, which showed a small change between seasons (< 9 %). Clones T1, T23, and T24 showed the highest yields; similar to those observed in T8, T12, T14, T17, and CCN 51 (p < 0.05).
Table 5 Annual, rainy and dry season fresh cacao beans yield for Ecuadorian Nacional cacao clones. Mean values of 10 plants for a three- year period ± standard error.
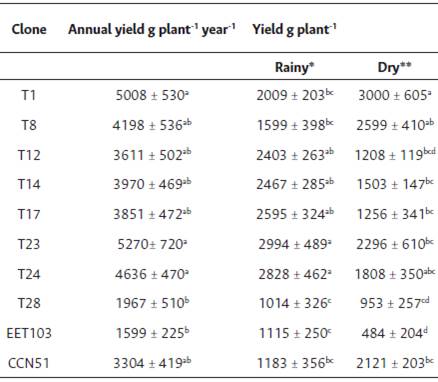
*(December-May); **(June-November). Similar letters in the same column are not significant (p < 0.05) according to Tukey's test.
All clones had higher yields during RS of the year with the highest rainfall recorded (2012). During 2014, the driest year, all clones decreased yields in RS. Clones T1, T8, and CCN 51 increased their production in the drought period as the year was drier, while the other clones did not show that trend (Figure 4). No significant correlations were found between yield and physiological traits.
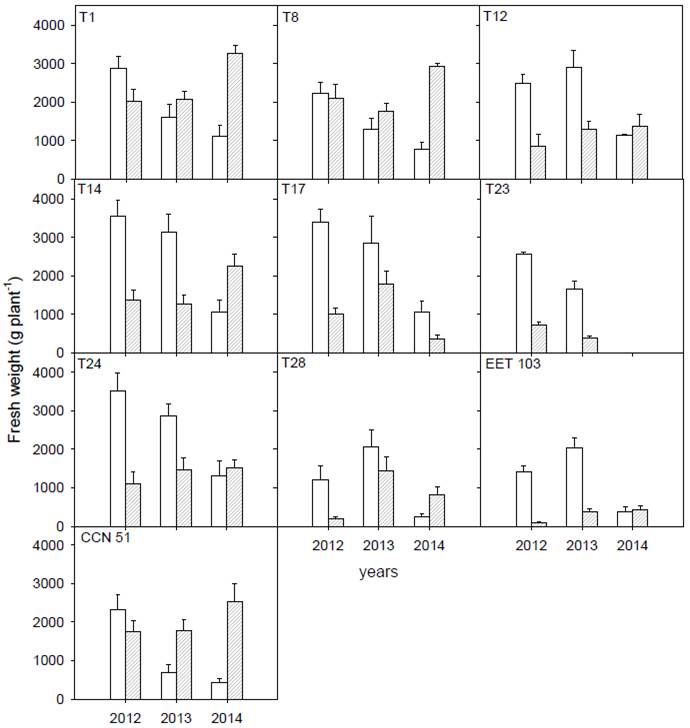
Figure 4 Bean fresh weight per pLant over three years of 10 seven-year-old clones of Ecuadorian cacao measured during dry (striped bars, May thru October) and rainy (open bars, November thru April) seasons. Data of T23 for 2014 was collected but was removed from the analysis due to data inconsistency.
Discussion
Soil moisture in the field after 120 days without rain was close to the value obtained at -456 KPa in the laboratory, indicating an intermediate water availability between field capacity and the wilting point. Rains lasted until June 23 in 2015, which is not usual for the region. Lower SWC may probably be obtained when the rains end in May as it usually occurs. As was expected, drought in the Ecuadorian coast caused a reduction in A and E as a consequence of a decrease in leaf RWC, which varied among the cacao clones studied. Stomatal conductance showed differences among clones; but, no significant reduction in gs mean was observed between seasons. During DS, NPQ increased in most clones as an effective mechanism to dissipate excess heat to avoid damage to the photosynthetic machinery. Clones showed differences in macronutrient content and drought reduced Ca and Mg content in almost clones. The T1 and T8 clones can be selected for future drought-tolerance breeding programs, since no significant changes in nutrient content was found during the year and due to its high yield during DS. Drought caused reduction in an annual yield in almost clones, however T1, T8, and CCN 51 showed higher yields during DS.
Gas exchange
There were differences in gs between clones for both seasons, and a decrease in A of 30 % during drought, however the relationship between gs and A was low (r2 = 0.10). The little variation between seasons found in gs agrees with that obtained in the same region for the cacao Nacional clones under field conditions (Jaimez et al., 2018)). It seems that, in the same study area, gs is regulated largely by VPD; spite lower SWC, low VPD due to high humidity (above 65 %) and cloudy days during DS little affect gs (Jaimez et al., 2018). Maximum VPD are between s1.3 and 1.6 KPa in the region, suggesting that gas exchange in cacao is not limited by stomatal closure. Variations found in gs between clones during drought show that there are different levels of sensitivity to water deficit to conserve water and different mechanisms used by cacao clones to tolerate water deficit (Lahive et al., 2018). Indeed, reduced A was correlated to a reduction in the RWC. The RWC obtained for RS were similar to those found under field conditions by Avila-Lovera et al. (2016). In cacao seedlings was reported a RWC of 90 % in well watered plants; after 60 days without irrigation, RWC gradually decreased to 50 % (Almeida et al., 2002). Reductions in RWC affects several metabolic processes, such as uncoupling of the photosystems which lead to reactive oxygen species (ROS) production, reduction or inhibition of enzymes and ATP synthesis and an increase in photorespiration (Lawlor & Tezara, 2009; Farooq et al., 2012). This may lead to a reduction in photoassimilates which, depending on its distribution to the different parts of the plant, specific to each clone, could negatively affect yields to a greater or lesser extent.
It seems that non-stomatal factors could have played a major role affecting A under field conditions in the central area of the Ecuadorian coast, especially during drought. Similarly, Tezara et al., (2020) have reported that metabolism was inhibited by drought in Trinitarios and Modern Criollo cacaos during DS. In Ghana, cacao seedlings of several clones showed lower gs in DS compared to RS (Acheampong et al.,2013). Stomatal closure was also reported during periods of drought in field conditions in Criollo cacao cultivars in Venezuela (Araque et al., 2012; Avila-Lovera et al., 2016; De Almeida et al., 2016; Tezara et al., 2020). However, Avila-Lovera et al. (2016) also found clones with similar gs during dry and rainy seasons and that maximum A values did not correspond with the highest gs values in the clones studied. The lack of a relations'hip between A and gs observed in this study has been reported previously in other cacao cultivars (Baligar et al., 2008).
Maximum A in cacao has been obtained at PFDs of 300-600 μmol m-2 s-1 (Almeida et al., 2014; De Almeida et al., 2018). In Ecuador, in the last twenty-five years, clones have been developed under cloud cover and high relative humidity in the coastal region and few plantations use shade. The exposure of cacao plants to full sun in the overcast coastal Ecuador has led to a greater efficiency of the photosynthetic machinery at PFDs above 400 μmol m- 2 s- 1, as recently reported by Jaimez et al. (2018) in Ecuadorian cacao clones, also in17 National clones from a selection of hybrids and three Criollos clones at the North of Esmeraldas province, Ecuador (Tezara et al., 2015) and in CCN 51 clone in Colombia (Suárez et al., 2018). The strategy of developing unshaded cacao plantations in the central Ecuadorian coast is apparently a good one, since A would otherwise be lower due to limitations of both PFD and water during drought. This environmental condition is very different from other regions where the dry period is characterized by high radiation and lower relative humidity and temporary or permanent shades are provided by different species according to the locality (Acheanpong et al., 2018; Araque et al., 2012).
An average decrease of 33 % in A obtained in this study in the late dry period should also certainly affect yield in some cacao clones. In contrast, full exposure is not suitable for Criollo cocoa, as high PFD caused photoinhibition of PSII, which may result in lower resource use efficiency and reduced tree lifespan (De Almeida et al., 2018). Therefore, supplementary irrigation should be initiated at least 100 days after the start DS in the region. It is known that cacao yields are strongly affected by lower SWC (Moser et al., 2010), which may be due to its shallow root system that reaches down to 0.8 m deep, and the long drought periods limit water uptake at these depths.
The WUE was higher during RS, in agreement with long-term WUE (higher carbon isotope composition; 513C) found in Criollo cacao (Avila-Lovera et al., 2016). It seems that water deficit tolerance in cacao was not regulated by the stomatal mechanisms under ambient of high humidity levels. However, T23 displayed a major and significant WUE during drought at high PFDs. This optimization, which involves water loss at critical times of water availability, should be considered in the selection of this clone for breeding programs.
Photochemical variables
Dry season did not affect ΦPSII in any cacao clones studied, suggesting that drought does not caused damage to photochemical activity. Similar results have been reported in Criollo and Trinitario cacao under field conditions (Araque et al., 2012; De Almeida et al., 2016). In contrast, photochemical activity in 31 Venezuelan cacao clones were reduced during drought, suggesting that metabolism was inhibited (Tezara et al., 2020). In Ecuadorian cacao clones studied, energy thermal dissipation through increased NPQ helps to prevent the generation of ROS that result from excess light absorption during drought, as have been reported before (Jaimez et al., 2018, Suárez et al., 2018; De Almeida et al., 2018; Tezara et al., 2020). An increase in NPQ in cacao plant subjected to high PFDs and drought represent an efficient mechanism of photoprotection to avoid photo damage of PSII.
Macronutrient uptake
Leaf macronutrient contents were significantly different between Ecuadorian clones and affected by drought. Similarly, differences in leaf nutrient content in seedlings of cacao clones under controlled and field conditions submitted to water deficit have been reported (Santos et al., 2014; Puentes-Paramo et al., 2016). In our study only three clones showed significant changes in N content between seasons. It is possible that significant increase in leaf N found in T17 and CC N51 during drought may be related to a rapid accumulation of amino acids due to a low rate of protein synthesis (Viets, 1972). In Criollo and Trinitarios cacao, drought in field conditions induced a lower leaf N content (Araque et al., 2012; Avila-Lovera et al., 2016; Tezara et al., 2020). Lower A has been associated with a reduction in leaf N content (Avila-Lovera et al., 2016; Tezara et al., 2020). Leaf P content of the clones studied remained unchanged, which agrees with the report in Criollo cacao cultivars in Venezuela (Araque et al., 2012). Apparently, the uptake of P in cacao is little affected by drought in field conditions; however, the seedlings of some cacao clones have reduced P contents under drought conditions (Santos et al., 2014). Several Forastero cacao showed a significant increase in P content during drought, while in other clones was unchanged (Almeida et al., 2002). As a consequence, it appears that young cacao plants are more sensitive to drought and therefore experience changes in P uptake which differs among clones. The 4 months period without rain in which clones showed no change in leaf P content suggests that the roots of mature Ecuadorian cacao plants have the capacity to absorb P and its uptake does not depend on the duration of water deficits in the Ecuadorian coastal region.
In spite of lower E the long-duration of water deficit induced a significant increase in leaf K content in six clones studied; this trend has been reported before in cacao seedlings (Almeida et al. 2002); suggesting that K may play an important role in osmotic adjustments during drought.
Few studies have been carried out on Mg uptake under drought conditions in cacao. In the Ecuadorian cacao clones studied, the gradual dehydration of leaves led to a decrease in Mg which varied among clones. Physiological responses to Mg deficiency are complex for example, N metabolism is affected and the sucrose entry to phloem is interrupted but others processes are not yet known (Guo et al., 2016). In Citrus reticulata has been shown that Mg deficiency lead to a decrease in electron transport which caused a decline in A (Tang et al., 2012). Santos et al. (2014) have proposed that Mg content should also be a criterion for the selection of drought tolerant cacao cultivars since its deficiency leads to lower rates of A, as was found in our study. Even though Ca was lower in drought-stressed plants of different clones, only two clones showed a significant decrease. Several problems could be caused by Ca deficiencies, such as cell and membrane deterioration and consequent loss of turgor (Rouphael et al., 2012), which is necessary to keep stomata open. Gilliham et al. (2011) proposed a model in which movements of Ca influence the flow of water through plants where a dynamic equilibrium can be established or produce oscillations that lead to a balance of the water flow between cells.
Yield
The physiological traits evaluated do not directly influence yield, since not correlation between A and yield was found and probably the combination of several traits of them influence yield. Results of cacao bean fresh weight showed that clones could be divided into three groups based on their production during the different seasons: six clones (T12, T14, T17, T23, T24, and EET 103) that maintained higher yields during RS, three clones (T1, T8, and CCN 51) had higher yields under drought conditions and T28 had similar yield in both seasons. T1 and T8 come from the same cross (CCN 51 x EET-233), that likely to lead to clones with higher yield in the dry period. Additionally, the highest-yielding clones in the drought period showed the highest yields in the year of least precipitation in DS (year 2014). While higher-yielding clones during RS kept yields in the dry period in the year of lower precipitation. The yields of most clones in the rainy period were associated with the total amount of precipitation of each year. For example, in a dry year (around 1550 mm total precipitation) the yields in RS reach 1450 g fresh weight per plant, while in years with total annual precipitation between 2050-3200 mm, the yields in RS reach values between 3100-3500 g of fresh weight per plant. Cacao growth rates and yields have previously been related to the amount and distribution of rainfall (Moser et al., 2010); furthermore, yield has been related to soil water storage capacity. The length of drought periods was variable among years and is one of the main limiting factors that affect the stability of cacao yield (Carr & Lockwood, 2011).
Lahive et al. (2018) highlighted that little is known about the processes affected by water deficit in the early stages of development of cacao pods. In Ecuador, the period of greatest production occurs between October and December. This means that the first stages of pod formation in a large number of farms must occur in the first three months of DS (July-August). However, soil water availability of this period does not strongly limit A. It seems that clones with higher yields during DS showed different strategies for assimilate accumulation. It is probable that pod growth rates are low due to gradual reduction of available soil water; however, it seems that a greater amount of assimilates are allocated to pod growth during DS in an exceptional region of the world where cacao can be cultivated without irrigation for up to 140 days without rain.
The high yields in two Nacional clones (T1 and T8) offers important strategic alternatives because farmers can choose to grow a combination of these new released Nacional clones and thus maintain relatively high yields throughout the year, even in dry years.
Conclusion
In conclusion, in the case of Ecuadorian cacao clones growing in the central coastal regions where overcast and low evaporative demand predominate, gs shows differences among clones but it was relatively constant during drought, suggested that non-stomatal limitations also lead to a reduction in A during drought. During DS, decrease in RWC produce reduction in A and E about 33 and 22 %, and decreases leaf Mg content and it is likely that these changes lead increases in NPQ as a photoprotective mechanism and increases in K that could be related to a greater osmotic adjustment.
The differences in yield between clones are related to the dynamics of pod production in drought and in RS and the total rainfall. The yield of the clones is affected negatively by drought but the level of affectation depends on the intrinsic capacity of distribution of assimilates towards pods and seeds that each clone has.