Services on Demand
Journal
Article
Indicators
-
Cited by SciELO
-
Access statistics
Related links
-
Cited by Google
-
Similars in SciELO
-
Similars in Google
Share
Ingeniería e Investigación
Print version ISSN 0120-5609
Ing. Investig. vol.30 no.2 Bogotá May/Aug. 2010
Treatment of COD analysis liquid wastes generated in environmental laboratories
Tatiana Mañunga1, Héctor Mario Gutiérrez2, Jenny Alexandra Rodríguez Victoria3 and Alexander Villarreal Díaz4
1 Sanitary Engineer, Universidad del Valle, Cali, Colombia. M.Sc. Student,Universidad del Valle, Cali, Colombia. Research Assistant, Grupo ECCA,Universidad del Valle. Cali, Colombia. Universidad del Valle. Cali, Colombia. tatianam@univalle.edu.co 2 Chemical. M.Sc., Universidad del Valle, Cali, Colombia. Assistant Professor,School of engineering, Universidad del Valle, Cali, Colombia. hector.gutierrez@correounivalle.edu.co 3 Sanitary Engineer, Universidad del Valle, Colombia. M.Sc. y Ph.D., Universidad Sao Paulo, Sao Paulo, Brasil. Associate Professor, School of engineering, Universidad del Valle, Cali, Colombia. jenny.rodriguez@correounivalle.edu.co 4 Sanitary Engineer, Universidad del Valle, Cali, Colombia. Specialization Student, Universidad del Tolima, Colombia. Planning Coordinator works,Centroaguas S.A. ESP. alevidaz@univalle.edu.co.
ABSTRACT
COD analysis is often carried out in environmental labs. Its wastes are considered hazardous due to the content of metals such as Cr, Ag and Hg; treating these wastes is considered complex and expensive. The experimental results of metal ion precipitation in COD wastes with affordable chemical products are reported in this work. Cr (VI) was chemically reduced by adding 200 mg.L-1 of glucose to Cr (III). Final Cr(VI) concentration was less than 0.5 mg.L-1. Cr (III) was precipitated as a metallic hydroxide by adding NaOH and Ag was reduced to less than 0.2 mg.L-1 by adding 2 g.L-1 of NaCl. Hg was reduced to less than 0.005 mg.L-1 with 10 g.L-1 of FeS. The proposed reduction-precipitation methodology allowed minimising the liquid residue's hazardous characteristics so that it complied with the maximum allowable values established in Chapter 6, Article 74 of Decree 1594/1984 that regulates the use of water and liquid residues.
Keywords: parallel, control, ternary system, column, reactor.
Received: april 12th 2009
Accepted: may 10th 2010
Introduction
Different physicochemical analysis based on chemical substance use and operations producing hazardous residues are usually carried out in research and educational environmental labs. Chemical oxygen demand (COD) analysis is conducted on a regular basis as it allows determining organic matter contamination in water. Although COD is an extremely useful analysis, it produces a liquid residue which is considered hazardous and harmful to the environment due to low pH values and high silver (Ag), mercury (Hg) and chromium (Cr) concentration (Hendrickson et al., 1984). High concentrations of Hg and Cr (VI) have been associated with toxic and carcinogenic characteristics (EPA, 2008).
Volume and toxicity reduction of liquid waste is the main objective of hazardous waste treatment and it is usually carried out by physicohemical, biological and thermal processes (Márquez, 2004).Reduction-precipitation is a physicochemical treatment process based on the formation of a sparingly soluble molecule due to very low Kps values (Teg Leong et al., 2001). However, Cr (VI) concentration must be decreased to avoid this ion interfering with the reduction- precipitation process. An easy way to decrease Cr (VI)concentration is by adding an easily oxydable organic material to the liquid waste thereby facilitating the reduction of this metal to its trivalent state (less reactive). It has been shown that by adding 50 mg.mL-1 concentration fructose at room temperature and 2 pH that Cr (VI) final concentrations ranging from 0.23 to 0.27 mg.L-1 can be obtained in 60 minutes (Balderas et al., 2007).
After decreasing Cr (VI) concentration, COD residue treatment is carried out through a series of precipitation reactions in which an insoluble salt is formed from the ions present. Silver chloride (1.8 x10-10Kps) is formed from silver by adding sodium chloride (common table salt) at 1.97 g.L-1 concentration and it is insoluble in both acid and alkali (Márquez, 2004). Cr (VI) reduction of around 99% has been reported (López, 2006).
Likewise, mercury can be reduced/precipitated as mercury sulphade(HgS) which is insoluble, even at room temperature, and has a Kps of 4x10-53, so that concentrations in the order of 10-22 to 10-7 g.L-1 can be obtained (Banfalvi, 2006). 99% reduction of mercury ions has been obtained by adding 10 g.L-1 of iron sulfide (FeS) (López,2006).This precipitation takes place at pH values lower than 3 and produces hydrogen sulphide (H2S) which is volatile, toxic, corrosive and has a strong odour (Holm, 1996).
Most metals tend to be more available (dissolved) at acid pH and, in the case of Cr (III), increased solution alkalinity decreases mobility,precipitating as chromium hydroxide. Cr (III) reductions higher than 98.5% have been obtained at pH values ranging from 8.0 to 9.0 (Cabrera and Martínez, 2007) because, in this range, Cr (OH)3 has a minimum 2.9x10-29 Kps (Márquez, 2004). However,Cr (III) precipitation with sodium hydroxide (NaOH) produces heat due to the exothermic reaction and toxic gases thereby requiring strict safety measures.
The cost involved in the chemical treatment can become significantly reduced by using readily available reactants due to their common use in chemical analysis. Leong (Leong et al., 2001) reported an operational cost of 19.95 Bath (Thai currency) for a litre of COD residue treated by reduction-precipitation (around 1,275 Colombian pesos in 2008). The cost was mainly attributed to the reactants used (reductive and precipitation agent) and the solidifycation and disposal of the solid residue so produced.
The objective of this study was to present an efficient and environmentally sound methodology for treating COD residues generated in environmental analysis labs. The methodology proposes a sequence of chemical reactions in which heavy metals are eliminated as hazardous solid residues; this is supported by previous results regarding the selective precipitation of Cr, Ag and Hg ions and assessed through lab experiments leading to establishing optimal dosage and settling time.
Materials and Methods
The liquid residues from COD analysis used in this study were obtained from the Universidad del Valle's Sanitary and Environmental Engineering Department's environmental technology lab (sample 1), watier and environmental residues lab (sample 2) and environmental chemistry lab (sample3). All the experiments were carried out with constant stirring to guarantee good mixing in the reaction vessel. The upper layer and sludge formed in each precipitation experiment were filtrated with a fast filtration media (Ø=125 mm) to provide selective separation of Ag, Hg and Cr\ ions. The solutions and dilutions were prepared with distilled and acclimatised water at 25 ±1 °C.
Residue characterisation
The COD liquid residues samples had Cr, Ag and Hg concentration higher than the maximum allowable limits for liquid discharges according to Colombian Decree 1594 /1984 (Minambiente,1984) regulating the use of water and liquid residues. Table 1 shows the initial concentrations of metals in the samples.
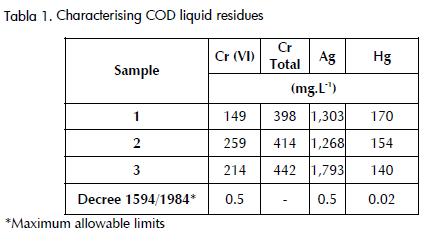
Chemical precipitation
The sequence of Ag, Hg, and Cr ion precipitation was carried out as follows.
Reduction of Cr (VI) to Cr (III)
Cr (III) was reduced with a 50% concentration glucose solution (commercial sugar). Glucose solution dosages of 20, 50, 100, 200 and 250 mg L-1 were added to 500 mL volume of COD residue sample. The Cr (VI) reduction reaction and Cr (III) formation was followed through the absorbance of the solution at different times. The wavelengths for indirectly determining the Cr (VI) and Cr (III) concentration were 420 nm and 620 nm, respectively. Cr (VI) reduction was verified by initial and final concentration according to Standard Methods 3500 Cr-B (APHA, 2005)
Silver precipitation (Ag)
Once chromium had been reduced, 2 g of sodium chloride (NaCI) per litre of residue was added to the solution and stirred for 10 minutes. This dosage has been reported by Lopez (2006) to guarantee the formation of AgCl2 due to the excess of Cl- in the solution. After stirring up the sample, the precipitated sludge volume was measured with an Imhoff cone and the supernatant was measured for final Ag concentration.
Mercury precipitation (Hg)
10 g of iron sulphide (FeS) per litre of residue was added to the free Ag ions in the supernatant. This dosage allows HgS formation (López, 2006). The mixture was stirred for 2 h, precipitated sludge volume was measured with an Imhoff cone and the supernatant was measured for final Hg concentration.
Cr (III) precipitation
The supernatant from the Hg precipitation experiment was subjected to Cr (III) precipitation at different dilutions (1:10, 1:5, 1:4, 1:3 and 1:2). Under constant stirring, 30 mg of trisodium phosphate per litre of solution was added to the solution to increase alkalinity. The pH was adjusted to 8.5 ± 0.5 by adding a concentrated 50% solution of commercial caustic soda (NaOH). The precipitated sludge volume was measured with an Imhoff cone. This step allowed selecting the best dilution that produced the lowest Cr (III) concentration in the shortest precipitation time and in safe operational conditions (due mainly to the high temperatures produced by adding NaOH).
A considerable amount of suspended solids were observed during this experiment. Precipitation tests with ferric chloride (FeCl3) were carried out to improve the quality of the supernatant and decrease precipitation time.
Co-precipitation of Fe and Cr (III) with NaOH and FeCl3
The filtrated upper layer from the mercury precipitation, diluted with the optimal ratio found in the previous experiment, was subjected to a jar test to determine optimal FeCl3 dosage. The jar tests' dosages and operational conditions are shown in Table 2. The pH was adjusted with 30 mg of trisodium phosphate and a 50% NaOH solution with fast mixing.
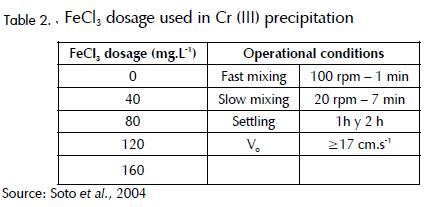
By-products: The sludge generated in each precipitation step was dehydrated at room temperature. These residues were segregated, packed, identified and stored according to Decree 4741/2005 (Minambiente, 2005) regulating the handling of hazardous residues. The ratio between generated dry residue and COD-treated residue volume was determined.
Analytical methods: Residue characterisation, taking and presserving samples was done according to the Standard Methods for Examination of Water and Wastewater (APHA, 2005). The parameters analysed in this study were pH, heavy metal concentration (Ag, Hg and Cr) and settling solids. Ag and Cr determination was carried out by flame atomic absorption spectrometry and mercury was determined by the cold vapour technique.
Results and Discussion
Cr (VI) to Cr (III) reduction
It was observed during the experiment that 20 and 50 mg.L-1 glucose dosages were not effective for reducing Cr (VI), whereas, a reduction was observed after 20 minutes reaction with 100 mg.L-1 dosage. However, this amount was not enough to accomplish complete reduction to Cr (III). Dosages equal to or higher than 200mg.L-1 added in time t=0 allowed Cr (VI) concentrations lower than 0.5 mg.L-1 to be obtained (Figure 1), indicating that most of the Cr (VI) became reduced to Cr (III). Dosages higher than 250mg·L-1 showed a white precipitate due to the excess of organic material addded.
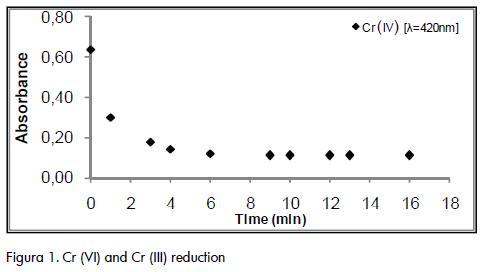
The reaction time in which maximum Cr (VI) reduction was achieved varied and depended on several factors such as the samples' source. In the case of sample 2, whose initial Cr (VI) concentration was 259 mg.L-1, a 25 minute contact time was required to achieve 99.9% reduction, whereas sample 3, whose initial Cr (VI) was 214 mg.L-1, required 50 minutes time to obtain a similar reduction. A final 0.45 mg.L-1 Cr (VI) concentration was obtained for sample 1 in 33 minutes (99.7% reduction).
Due to the variable characteristics of the COD residue samples and the experimental results, it was determined that a 50 to 60 minute reaction time was needed to obtain a final Cr (VI) concentration of less than 0.5 mg.L-1 with a dosage of 200 mg·L-1 of 50% glucose with constant stirring.
Silver precipitation (Ag)
The immediate formation and coalescence of large particles was observed during silver precipitation by adding NaCl. Fast settling of the heavier solids was observed when the mixture was placed in the Imhoff cone, leaving a suspension of fine particles that required a longer time to precipitate. After several replicate experiments, it was observed that phase separation was obtained after 24 hours of settling and that the supernatant had an average silver concentration of less than 0.2 mg·L-1, which was below the maximum allowable limit established by Decree 1594/1984.
Silver reductions of around 99.9% were obtained by adding 2 g.L-1 of NaCl independently of initial Ag concentrations. Figure 2 shows the Ag concentrations for the samples analysed here. An average 7 mL of sludge per litre of sample was produced during this step of the process and was then stored in glass recipients due to its corrosive characteristics (pH < 1 units).
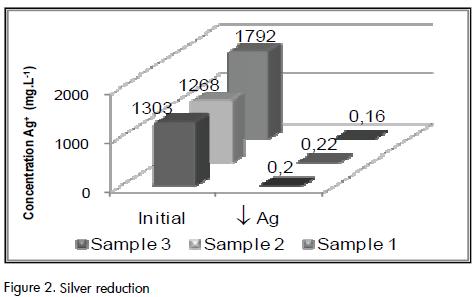
Mercury precipitation (Hg)
The analysed samples produced Hg concentrations of less than 0.005 mg.L-1 by adding 10 g FeS er litre of residue and 24 h o precipitation (i.e. 99.99% reduction). Around 15 mL of sludge was generated per litre of treated sample. Figure 3 shows the initial and final mercury concentrations in the analysed samples. FeS addition increased Fe concentration in the upper layer that presented (together with the sludge) acid characteristics (pH< 0.1 units).
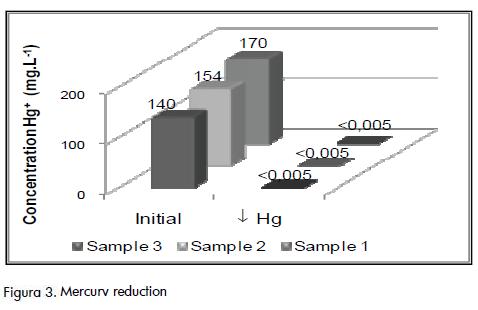
Chromium precipitation
Difficulty in adjusting the pH to 8.5±0.5 units proportional to sample dilution increase was observed during this essay. 50% NaO dosages higher than 270 mL (135 g.L-1) increased sample temperature to over 65o°C for dilutions 1:2 and 1:3 and it became necessary to decrease the temperature to 35o to continue pH adjustment and decrease the formation of toxic vapours produced during the reaction. Alternatively, crystals were formed on the precipitated sludge by adding 135 g.L-1 NaOH dosages producing a white layer in the bottom of the flasks, indicating that the amount of caustic soda was higher than that theoretically required to form the insoluble chromium hydroxides.
It was observed in each dilution that the particles (Cr hydroxides) coalesced more at pH values near to 8.5 ±0.5 due to the proxymity to minimum solubility point. A settling time of 24 h was necessary to obtain a clear supernatant and a compact sludge. After this time, Cr concentration became less than 0.1 mg.L-1, thereby complying with Colombian regulations.
The experimental results showed that a 1:4 dilution was suitable for precipitating Cr (III) because it allowed a better pH adjustment with NaOH dosage ranging from 139 to 108 g.L-1. The maximum temperature was 47.8°C and Cr (III) reduction percentage was 99.9%. Fe reduction was 99.3% with an average final concentration of 9.71, 9.86 and 9.45 mg.L-1 for samples 1, 2 and 3, respectively.
Fe and Cr (III) co-precipitation with NaOH and FeCl3
Final Cr (III) concentration in the jar test after 1 hour of precipitation was less than 0.1 mg.L-1, which indicated that this was the time required to reduce Cr by more than 99.9% (See Figure 4). This result agreed well with the findings reported by Villarreal and Mañunga (2008) who showed that chromium precipitated as a metallic hydroxide at pH values ranging from 8 to 9.
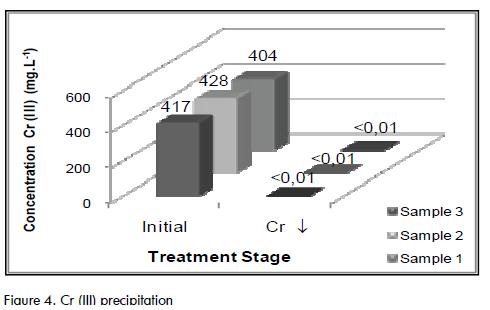
Bearing in mind that the same result was obtained with no addition of ferric chloride, it was inferred that this substance had no effect on Cr (III) precipitation in the experimental conditions used here. This result showed that the ferric hydroxides (Fe(OH)3) at pH 8.5±0.5 did not efficiently precipitate in the assessed time, requiring a time as long as that observed in the previous experiment (24h) or that it was possible to reduce this to 99% in a shorter time by improving stirring conditions (2 h) (See Figure 5). This could be attributed to the fact that Fe, as a metallic hydroxide, reached its minimum solubility at pH 4 (Márquez, 2004).
The increase in Fe concentration during Hg precipitation (as observed in Figure 5) was related to the reactive used to precipitate Hg. Although it was a disadvantage of this step, it did not affect the final quality of the effluent since it was possible to remove Fe during Cr (III) precipitation. The ferric chloride in the Fe precipitation was effective only in the sample having a 80 mg.L-1 dosage of FeCl3 2% and final Fe concentrations were equal to 4.88 mg.L-1.
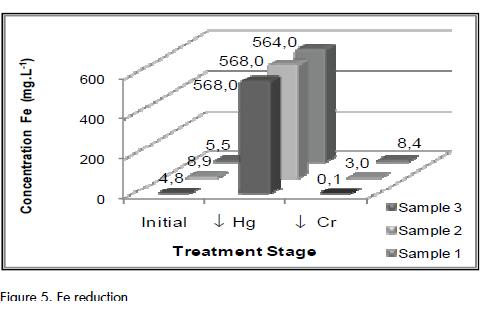
By-products
Sludge having the residue's characteristics was produced during each precipitation stage. The sludge generated during Ag and Hg precipitation had pH lower than 2, these being toxic and corrosive characteristics of COD residue. The sludge generated during t Cr (III) precipitation showed a pH ranging from 8 to 9 because the residue had been previously neutralised with NaOH.
Table 3 shows the amount of sludge generated in each precipitation step per litre of treated COD residue. The results indicated that there was an important difference in the residue from the Cr precipitation experiment which could have been attributed to the effect of the source of the samples in the characteristics of the generated COD residue as Cr (VI) reduction during the COD experiments depended on the amount of oxydable organic material in the sample.
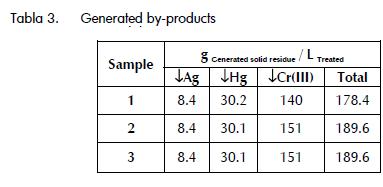
It was also observed that the high production of residue during Cr precipitation was a consequence of the high concentrations of this metal in the initial residue.
Conclusion
Glucose added as easily oxydable organic material allowed reducing Cr (VI) to less than 0.5 mg.L-1, in a 60 minute reaction time. Ag and Hg ions were precipitated to obtain concentrations less than 0.2 and 0.005 mg.L-1, respectively. Each of the obtained conversions was less than the maximum allowable limit for liquid discharges stipulated in Decree 1594/1984.
The simulation of fast and slow mixing conditions during Cr (III) precipitation produced Fe and Cr metallic hydroxides having improved sedimentation characteristics, reducing settling time to h with Cr concentrations in the upper layer of less than 0.1 mg.L-1.
The proposed methodology for treating COD residues generated in environmental labs is a feasible and environmentally sound alternative allowing institutions to comply with regulations regarding maximum allowable heavy metal discharge in liquid waste.
Reduction and selective precipitation concentrates the ions present in COD liquid residues into a solid residue; the by-product is a sludge which can be raw material for an Ag, Hg and Cr recovery/extraction process.
American Public Health Association (APHA)., Standard Methods for the Examination of Water and Wastewater., 20th edition, Washington, DC. USA, 2005. [ Links ]
Balderas, P., Hernández, C., Roa, G., Gómez, R., Barrera, C., Reducción de Cr (VI), utilizando Fructuosa, Glucosa y Sacarosa., Facultad de Química., Universidad Autónoma del Estado de México Paseo Colón, México, 2007. [ Links ]
Banfalvi, G., Removal of insoluble heavy metal sulfides from water., Chemosphere, Vol. 63, 2006, pp.1231-1234. [ Links ]
Cabrera, L., Martínez, J., Reducción de cadmio y cromo de aguas residuales de acabados metálicos por precipitación química., Instituto de Ingeniería, UNAM, México, 2007. [ Links ]
EPA, E-CFR Electronic Code of Federal Regulations, Title 40: Protection of Environment., Part 268-Land Disposal Restrictions., 2008. [ Links ]
Hendrickson, K. J., Benjamin, M. M., Ferguson J. F., Goebel, L., Removal of Silver and Mercury from Spent COD Test Solutions., Journal Water Pollution Control Federation., Vol. 56, No. 5, 1984, pp. 468-473. [ Links ]
Holm, R. T., Treatment of Spent Chemical Oxygen Demand Solutions for Safe Disposal., WMRC Reports: Waste Management and Research Center, University of Illinois, 1996. [ Links ]
López, J., Separación de plata, mercurio y cromo de residuos provenientes de los análisis de demanda química de oxígeno DQO., Escuela de Ingeniería Química, Universidad del Valle, Cali, Colombia, 2006. [ Links ]
Márquez, F., Manejo Seguro de Residuos Peligrosos., Departamento de Ingeniería Química, Facultad de Ingeniería, Universidad de Concepción, Chile, 2004. [ Links ]
Ministerio de Ambiente, Vivienda y Desarrollo Territorial., Decreto 1594., Usos del agua y residuos líquidos, Colombia, 1984. [ Links ]
Ministerio de Ambiente, Vivienda y Desarrollo Territorial., Decreto 4741 de 2005., Prevención y manejó de los residuos o desechos peligrosos, Colombia, 2005. [ Links ]
Soto, E., Lozano, T., Barbarin, J. M., Alcalá, M., Reducción de metales pesados en aguas residuales mediante agentes químicos., Departamento de Ingeniería Química de la Facultad de Ciencias Químicas, UANL, México, 2004. [ Links ]
Tet Leong, S., Muttamara, S., Laortanakul P., Hung-Ta, L., Assessment of treatment alternatives for laboratory COD wastewater: a practical comparison with emphasis on cost and performance., 2001. [ Links ]
Villarreal, D. A., Mañunga, T., Tratamiento de residuos líquidos generados en análisis fisicoquímicos de muestras ambientales. Caso: laboratorios del Área Académica de Ingeniería Sanitaria y Ambiental de la Universidad del Valle., BSc Sanitary Engineering thesis, Universidad del Valle, 2008. [ Links ]