Services on Demand
Journal
Article
Indicators
-
Cited by SciELO
-
Access statistics
Related links
-
Cited by Google
-
Similars in SciELO
-
Similars in Google
Share
Ingeniería e Investigación
Print version ISSN 0120-5609
Ing. Investig. vol.35 no.1 Bogotá Jan.Apr. 2015
https://doi.org/10.15446/ing.investig.v35n1.46969
DOI: http://dx.doi.org/10.15446/ing.investig.v35n1.46969
F. D. Mayer1, G. F. Schettert2, R. J. S. Michel Júnior3, E. L. Foletto4 and R. Hoffmann5
1Flávio Dias Mayer. Chemical Engineer, Dr. Affiliation: Researcher, Department of Chemical Engineering, Federal University of Rio Grande do Sul, Brazil. E-mail: flaviodmayer@yahoo.com.br
2Giseane Fumagalli Schettert. Chemical Engineer, M. Sc. Affiliation: Researcher, Department of Chemical Engineering, Federal University of Santa Maria, Brazil. E-mail: giseanefs@hotmail.com
3Raul José dos Santos Michel Júnior. Industrial Engineer, M. Sc. Affiliation: Researcher, Department of Chemical Engineering, Federal University of Santa Maria, Brazil. E-mail: raulmichel@via-rs.net
4Edson Luiz Foletto. Chemical Engineer, Dr. Affiliation: Associate Professor, Depart- ment of Chemical Engineering, Federal University of Santa Maria, Brazil. E-mail: efoletto@gmail.com
5Ronaldo Hoffmann. Chemical Engineer, Dr. Affiliation: Titular Professor, Department of Chemical Engineering, Federal University of Santa Maria, Brazil. E-mail: hoffmann@ct.ufsm.br
How to cite: Mayer, F. D., Schettert, G. F., Michel Júnior, R. J. S., Foletto, E. L., & Hoffmann, R. (2015). Operation parameters of a small scale batch distillation col-umn for hydrous ethanol fuel (HEF) production. Ingeniería e Investigación, 35(1), 31-35. DOI: http://dx.doi.org/10.15446/ing.investig.v35n1.46969
ABSTRACT
Batch distillation applied to hydrous ethanol fuel (HEF) production on a small scale still requires operating conditions that ensure optimal top product quality and productivity. The aim of this study is to statistically validate a batch still through the employment of response surface methodology (RSM). Operational and productivity parameters were formulated in order to guarantee quality compliance with the legal requirements for the top product concentration, besides providing support information to control the production of HEF on a small scale. The reboiler control and dephlegmator temperatures maintained within the range of 97.5 to 99.5°C and 60 to 70°C, respectively, combined with a variable reflux ratio, was satisfactory in obtaining a top product concentration, in accordance with legal regulations, as well as high productivity. The results of this study may contribute to the assembly of a simple and low-cost batch distillation control system.
Keywords: Batch distillation, ethanol fuel, process optimization, factorial design.
RESUMEN
La destilación diferencial aplicada a la producción de etanol hidratado combustible (EHC) a pequeña escala todavía requiere condiciones de operación que aseguren la calidad del producto destilado y la productividad óptimas. El objetivo de esta investigación es validar estadísticamente la destilación diferencial mediante el empleo de la metodología de superficie de respuesta (MSR). Parámetros operacionales y de productividad se han formulado con el objetivo de garantizar el cumplimiento de la calidad de los requisitos legales para la concentración del producto destilado, además de proporcionar información de apoyo para el control de la producción de EHC a pequeña escala. El control de las temperaturas del rehervidor y deflegmador, mantenidos dentro del intervalo de 97,5 a 99,5 °C y de 60 a 70°C, respectivamente, en combinación con una relación de reflujo variable fueron satisfactorios en la obtención de una concentración del producto destilado en conformidad con las normas legales, así como una alta productividad. Los resultados de este estudio pueden contribuir al montaje de un sistema de control de destilación diferencial simplificado y de bajo costo.
Palabras clave: destilación diferencial, etanol combustible, optimización de procesos, diseño factorial.
Received: November 1st 2014 Accepted: February 4th 2015
Introduction
The production of hydrous ethanol fuel (HEF) on a small scale (farm scale) is an important contribution to the efforts to replace fossil fuels and enable the energy self-sufficiency of small farmers. In this regard, the quality of HEF produced is of great importance in its commercialization to third-party consumers since its standards are regulated by federal legislation. Beyond the issues of competitiveness and ongoing search for higher efficiency, quality assurance of fuel ethanol is crucial. In Brazil, such regulation is performed by the National Agency of Petroleum, Natural Gas and Biofuels (ANP), which states that the ethanol concentration of HEF should be between 92.5 and 93.8 wt% (95.1 to 96.0% v/v), as established by ANP resolution Nº 07 (ANP, 2014).
Batch distillation is an affordable technology employed in small-scale production because it has operational flexibility, handling different flows and concentrations of feed (Kister, 1992; Flodman & Timm, 2012), which is a key feature of small-scale production. However, this operating system has some problems inherent to its dynamic nature, making it difficult to control and optimize (Flodman & Timm, 2012). Thus, it has been difficult to obtain HEF from batch distillation in accordance with the quality standards required, especially regarding the ethanol concentration of top product. Moreover, another important variable in the process is productivity (liters of HEF per batch) since high volumes of ethanol and, concomitantly, the highest concentration possible is desired. The evaluation of a batch distiller for ethanol production on a small scale may demonstrate the inability of the equipment to meet quality requirements (low HEF concentration), as well as low productivity and negative energy balance (Mayer, Feris, Marcilio & Hoffmann, 2015). Some modification in conventional batch distillation — inverted batch distillation column, middle vessel column, and distillation column provided with a side withdrawal — were developed in order to reduce batch time, high temperature in reboiler, and operationality difficulties (Ahón & Medeiros, 2001; Demicoli & Stichlmair, 2004).
Usually, process dynamics on batch rectification use a control protocol based on constant or variable reflux ratio (Bauerle & Sandall, 1988; Kister, 1992). Other strategies for batch processing may be found (Peng, Li, Sheng, Song & Zhao, 2007; Modla & Lang, 2008; Flodman & Timm, 2012). If a constant reflux ratio is used, a higher initial top product concentration will be obtained, but the concentration will decay over time (Straatmann, Maciel & Secchi, 2008). In this case, it is possible to obtain a high recovery of the light product (ethanol) at the end of the process, but with a smaller average concentration. Alvarez et al. (2012) and Coelhoet al. (2012) present results that show an exponential decrease in ethanol concentration in the top product during batch distillation with a constant reflux ratio. Coelho et al. (2012) report that as the reflux ratio increases, the final concentration of the top product increases, as does the time required for the distillation due to the low hourly output. Zavala & Coronado (2008) used a constant reflux ratio aiming to maximize thermodynamic efficiency of batch distillation for a given top product concentration.
According to Diwekar (1996) there are three optimal control problems: a) maximum distillate problem; b) minimum time problem; and c) maximum profit problem. Zavala-Loría, Ruiz-Marín & Coronado-Velasco (2011) cited other two problems to optimize batch distillation control, including d) problem of minimum energy, and e) maximum thermodynamic efficiency problem. If the reflux ratio is continuously adjusted during the distillation process, then it may be possible to obtain a constant top product concentration while maintaining a constant rate of vaporization (L/V) or a constant distillate flow rate (Lopes & Song, 2010). At the end of the distillation, the top product collected will have a higher concentration, but the recovery will be lower. Lopes & Song (2010) report that, for the cases they analyzed, distillation conducted under variable reflux (keeping a constant rate of vaporization) is more profitable than that under constant reflux. Thus, since it is crucial to obtain a top product quality that complies with the established standards, the operating strategy that must be used is one that combines productivity and energy saving due to the use of a variable reflux ratio.
Based on these aspects, the main objective of this work is to statistically validate the reboiler and the dephlegmator temperature control strategy of a batch still, through a rotatable central composite design for the employment of response surface methodology (RSM). Such a formulation allows to an operational and productivity parameters that guarantee quality compliance with the requirements for the top product concentration and providing supporting information to control the production of HEF on a small scale, in accordance with the requirements established by the ANP.
Materials and Methods
Experimental tests were performed in a commercial distillation plant, in a batch still manufactured by Limana Poliserviços Ltda. (Figure 1a and 1b). It comprises a reboiler, 300 cm diameter-rectifying tower with 21 bubble plates, a dephlegmator, a translational chamber, a condenser, a degassing bottle, and a thermocouple in the reboiler and in the condenser. Dephlegmator is a device that acts as a partial condenser, resulting in extra contact stages during the distillation operation. The translation chamber coupled to the dephlegmator was the innovation introduced by this equipment design, ensuring an extra separation of ethanol / water mixture. The reboiler has a 1,700 L capacity and is heated indirectly with steam (heat transfer coil), where the steam flow controls and adjusts the temperature.
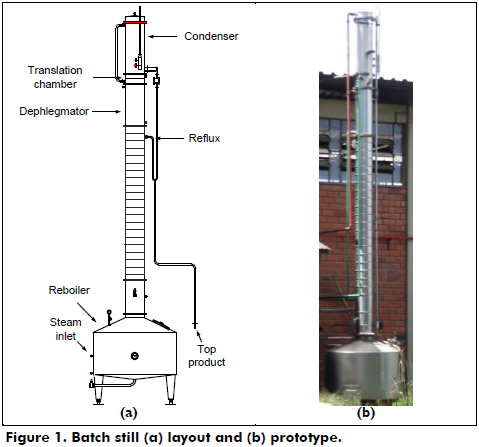
Water was used as a cooling fluid to control the temperature of the rising vapors in the dephlegmator and condenser. The translational chamber is a physical device that acts on the passage of ascending vapor and on its redirection, resulting in an increase in top product concentration. The concentration of the feed and product samples were analyzed on an ebulliometer, and the progress of distillation was monitored by an alcoholometer (densimeter) coupled to the top product output stream.
In each experimental test, the still was fed with 1,670 L of not filtered fermented wine made from sugarcane with an ethanol concentration ranging from 7.0 to 9.0% v/v. During the evaluation of the operationality and productivity performed to guarantee the top product concentration, the reflux ratio was manually adjusted, i.e., the reflux ratio was increased by decreasing the top product withdrawal, maintaining a constant rate of vaporization. The reflux ratio was continuously adjusted throughout the distillation operation in order to guarantee the maximum top product concentration. Previous experiments have shown that the precise control of the reboiler and dephlegmator temperatures, besides the continuous adjustment of the reflux ratio, optimizes the performance of batch stills. To evaluate these parameters, a rotatable central composite design was used to implement a response surface methodology. It is important to note that the batch time was not considered as a variable since it does not has the same importance as the top product concentration and productivity, when bioethanol self-consumption is considered.
In the factorial design, the temperatures of the reboiler (X1) and dephlegmator (X2) were defined as independent variables since they were the controlling tools of the distiller operation. Thus, the top product concentration and productivity were the dependent variables. Reboiler temperature (X1) was maintained constant via steam flow adjustment throughout the distillation, while the dephlegmator temperature (X2) was controlled by the flow of cooling water. The independent variables effect analysis was performed using a second-order factorial design, resulting in 11 experiments. Table 1 shows the matrix of the factorial design for the analyzed variables. The central points for X1 and X2 were 60 and 98 ºC, respectively, based on previous tests performed on the still.
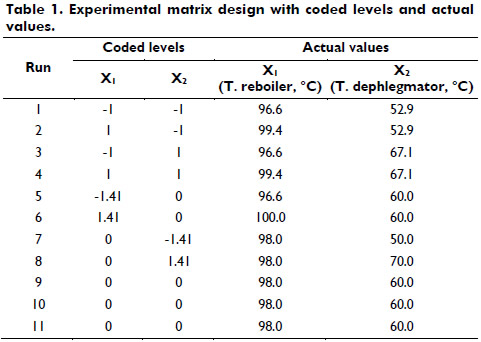
The second-order model with interaction terms for each dependent variable (Yi) was based on the method of nonlinear estimation. The empirical model, in terms of coded factors, is described by equation (1):

Where βi are the regression coefficients; β0 is a constant term; β1 and β2 are the linear effects; β12 is the interaction effect; β3 and β4 are the quadratic effects; and X1 and X2 are the independent coded variables (reboiler and dephlegmator temperature, respectively). The performance of model fit and the significance of the regression coefficients were evaluated by ANOVA. The response surfaces were prepared using the Statistica® version 7 software program.
Results and discussion
During previous testing, it was observed that the operation of the batch still was divided into three stages. In the first stage, which corresponded to the first 20 min, the productivity and the top product concentration were low. In the second stage, the productivity increased considerably, and the top product concentration increased to the range between 92 and 95% v/v. At this stage, the top product had such a condition that it could be used as automotive fuel. In the third and final step, the ethanol concentration in the top product fell to values below the desired range, near 20.0% v/v indicating that the process ended.
The discussed and presented results below refer to the second process stage, and are shown in Table 2. The top product concentration (Y1) presented in Table 2 are average values.
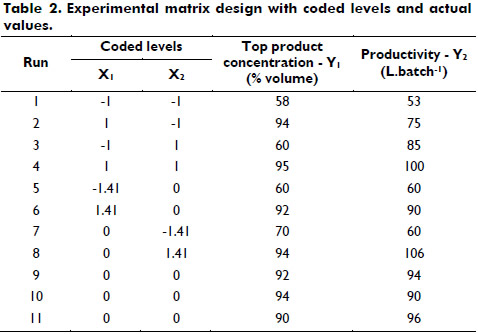
The dependent and independent variables were fitted to a second-order equation with interaction terms between the parameters [Eq. (1)]. Table 3 shows the regression parameters for each monitored response. The variance analysis of the top product concentration (X1), with a significance level of 10% (p <0.1), shows that the linear and quadratic terms of the reboiler temperature are significant. The interaction between X1 and X2 is also significant. The linear and quadratic terms of the dephlegmator temperature were not significant; therefore, a response surface could not be generated for the top product concentration. Regarding productivity, the ANOVA showed that all considered linear and quadratic effects for the reboiler and dephlegmator temperatures are significant, except for the interaction between X1 and X2.
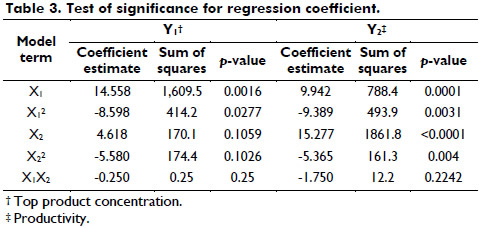
The effects of the factors studied in the system are demonstrated in the Pareto chart in Figure 2a and 2b. In accordance with the Pareto chart, for a confidence interval of 90%, it is possible to verify that the linear term for the reboiler temperature had the most significant effect on the top product concentration and the second most significant effect on the productivity. The effects of the quadratic term of the dephlegmator temperature on the top product concentration and productivity were the second and third most significant effects, respectively. The linear term related to the dephlegmator temperature did not have a significant effect on the top product concentration, but it had the most significant effect on productivity. The term representing the linear interaction between the temperature of the reboiler and the temperature of the dephlegmator were not significant with respect to the concentration of the top product or the productivity.
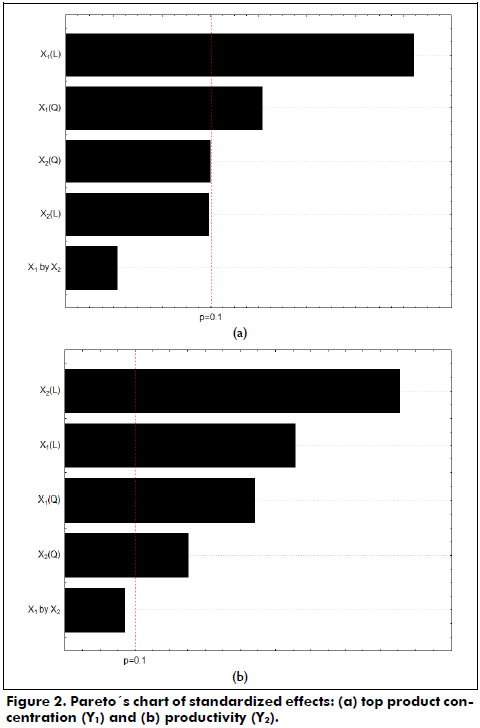
The regression Equations (2) and (3), that describe the empirical model (Eq. (1)) are based on data from Table 3, ignoring the terms that are not significant (p> 0.1). The empirical models in terms of the coded parameters are:

Where Y1 and Y2 are the top product concentration and productivity, respectively, and X1 and X2 are the coded values of the reboiler and dephlegmator temperatures, respectively.
The main effects on the responses were used to construct the response surface. It was not possible to construct the response surface associated with the top product concentration because the X2 interactions were not significant. This lack of significance indicates that for the control parameter X1 it is not necessary to adjust the dephlegmator temperature, possibly because the large number of separation stages. The total number of stages includes physical devices (bubble plates), the stage from partial reboiler, and additional stages provided by dephlegmator (partial condenser). When there are a large number of separation stages, the increase in internal reflux —due to increased condensation in the dephlegmator— has little or no effect.
Figure 3 shows the response surface for productivity (Y2) according to the reboiler (X1) and dephlegmator (X2) temperatures. It is observed that there is a region of higher productivity defined by the reboiler (between 97.5 and 99.5 °C) and dephlegmator (between 60 and 70 °C) temperatures.
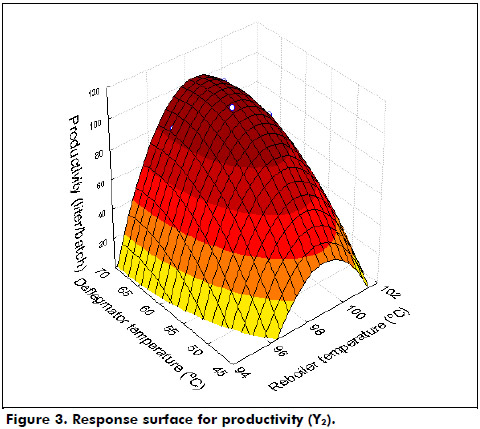
A higher reboiler temperature suggests a higher rate of vaporization of liquid, resulting in a higher vapor flow rate through the dephlegmator. Thus, the higher the dephlegmator temperature was, the greater the non-condensed vapor flow was, resulting in the peak in productivity observed when the reboiler temperature reaches 98.5 °C. Beyond that point, the productivity decreases considerably.
This reduction in productivity can be explained by the same arguments previously used to justify the achievement of peak productivity, which was increasing the ethanol/water mixture rate of vaporization in the reboiler. In a batch process, in which the reboiler temperature exceeds 98.5 °C and for a given dephlegmator temperature range, high vaporization of the ethanol/water mixture occurs. The higher the temperature is, the faster the vaporization of the light component (ethanol) will be, resulting in initially high top product productivity. However, during the course of batch distillation, there will be a gradual decrease in the ethanol content of the top product. To compensate for this decrease, the withdrawal of the top product should be reduced to increase the reflux ratio and ensure the desired concentration, sacrificing productivity.
It is also noted that in batch distillation there is a direct relation between the top product concentration and productivity due to reflux, i.e., if the reboiler and dephlegmator temperatures are adjusted to the ranges 97.5 - 99.5 °C and 60 - 70° C, respectively, it will be possible to obtain a top product with a high concentration and productivity by gradually adjusting the reflux ratio. This behavior is evidenced by analyzing the results of experiments 2, 4, and 6 presented in Table 2. These results define the region of maximum top product concentration and productivity, especially point 4, where the conditions allow for a top product, as well as high productivity, to be obtained in accordance with the national legal standards for commercialization of HEF. Therefore, the narrow range obtained for the reboiler and dephlegmator temperatures should be used as standard parameters in distillation operation in order to obtain HEF with legal conformity along as high productivity.
Figure 4 compares the predicted and experimental responses observed. The results indicate that the values calculated by the models described by Eq. (2) and (3) are in agreement with the experimental data with respect to both the top product concentration and the volumetric productivity of the distillate.
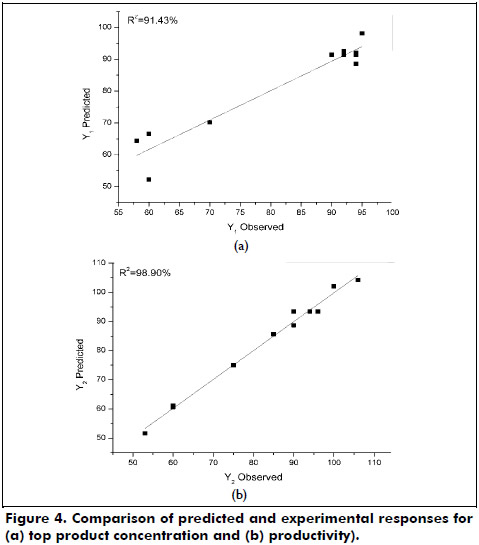
Based on the results it is clear that the control strategy is related with the adjustment of reboiler and dephlegmator temperature within the range of 97.5 - 99.5 °C and 60 - 70° C, respectively. It can be stated that the model described here represents the joint of the first three optimization problems described by Zavala-Loría, Ruiz-Marín & Coronado-Velasco (2011), namely the maximum distillate problem, the minimum time problem, and problem of maximum profit.
The model described in this work is applied to this designed distillation still, but the methodology to control the distillation process may be used in others batch stills. It is also important to note that the operational strategy described was successfully applied to variable ethanol concentration in the feed, ranging between 7.0 and 9.0% v/v, which is a common condition in small-scale ethanol production.
Conclusions
The operating conditions of batch distillation are decisive in guaranteeing the characteristics of a top product, particularly its concentration and productivity. The results of this study show that the control of the reboiler and dephlegmator temperatures of a batch still, in the range of 97.5 - 99.5 °C and 60 – 70 °C, respectively, coupled with the adjustment of the reflux ratio during the distillation process, guarantees the production of HEF with the concentration defined by Brazilian legislation while allowing for high productivity.
Therefore, a simplified batch distillation control system, whose purpose is to control the reboiler and dephlegmator temperatures as well as adjust the reflux ratio as a function of the concentration of the top product, can be envisioned. The concentration may also be inferred from the temperature of the hydroalcoholic vapor that reaches the condenser. Thus, by monitoring these three temperatures, it is possible to control the entire batch distillation process, making the control system both easy and inexpensive to operate.
Acknowledgment
Brazilian financial support provided by CAPES and CNPq is gratefully acknowledged.
Nomenclature
HEF hydrous ethanol fuel
X1 reboiler temperature (ºC)
X2 dephlegmator temperature (ºC)
Y1 top product concentration (%volume)
Y2 productivity (L per batch)
Greek Letters
β regression coefficient
References
Ahón, V. R., & Medeiros, J. L. (2001). Optimal programming of ideal and extractive batch distillation: Single vessel operations. Computers & Chemical Engineering, 25, 1115-1140. [ Links ]
Alvarez, M. E., Moraes, E. B., Rodrigues, J. C., Bonona, A. J., & Wolf-Maciel, M. R. (2012). Evaluation of the batch distillation process in the ethanol production. Computer Aided Chemical Engineering, 30, 632-636. [ Links ]
ANP (National Agency of Petroleum, Natural Gas and Biofuels). Resolution ANP n. 07, February, 09, 2011. Retrieved October 14, 2014 from http://www.anp.gov.br [ Links ]
Bauerle, G. L., & Sandall, O. C. (1988). Design of batch distillation columns for binary mixtures. Chemical Engineering Communication, 65, 155-160. [ Links ]
Coelho, T. C., Souza, O., Sellin, N., Medeiros, S. H. W., & Marangoni, C. (2012). Analysis of the reflux ratio on the batch distillation of bioethanol obtained from lignocellulosic residue. Procedia Engineering, 42, 131-139. [ Links ]
Demicoli, D., & Stichlmair, J. (2004). Separation of ternary mixtures in a batch distillation column with side withdrawal. Computers & Chemical Engineering, 28, 643-650. [ Links ]
Diwekar, U. M. (1996). Batch distillation: Simulation, optimal design and control. Washington D. C., USA: Taylor & Francis. [ Links ]
Flodman, H. R., & Timm, D. C. (2012). Batch distillation employing cyclic rectification and stripping operations. ISA Transactions, 51, 454-460. [ Links ]
Kister, H. Z. (1992). Distillation Design. New York, USA: McGraw-Hill. [ Links ]
Mayer, F. D., Feris, L. A., Marcilio, N. R., & Hoffmann, R. (2015). Why small-scale fuel ethanol production in Brazil does not take off? Renewable and Sustainable Energy Reviews, 43, 687-601. [ Links ]
Peng, B., Li, X., Sheng, M., Song, S., & Zhao, G. (2007). Chemical Engineering and Processing: Process Intensification, 46, 369-370. [ Links ]
Modla, G., & Lang, P. (2008). Feasibility of new pressure swing batch distillation methods. Chemical Engineering Science, 63(11), 2856-2874. [ Links ]
Straatmann, G. S., Maciel, M. C., & Secchi, A. R. (2008). Modeling of a batch distillation column for implementation in the EMSO simulator (in Portuguese). In Proceedings of the XVII Brazilian Congress of Chemical Engineering (2008). Recife, Brazil. [ Links ]
Zavala, J. C., & Coronado, C. (2008). Optimal Control Problem in Batch Distillation Using Thermodynamic Efficiency. Industrial & Engineering Chemistry Research, 47, 2788-2793. [ Links ]
Zavala-Loría, J. C., Ruiz-Marín, A., & Coronado-Velasco, C. (2011). Maximum thermodynamic efficiency problem in batch distillation. Brazilian Journal of Chemical Engineering, 28, 333-342. [ Links ]