Introduction
Rice is an essential commodity for more than 60% of the world population (Patel et al., 2010). The crop yield is influenced by climatic factors, physical and chemical soil conditions, water management, sowing date, variety, sowing rate, weed control, and fertilization (Yosef Tabar, 2013). Furthermore, climate change is considered a potential limitation for rice producers from the socio-economic point of view (Delerce et al., 2016). For Colombia, an increase of up to 7°C in temperature and a decrease in precipitation of 10% (IPCC, 2014) could generate crop yield instability between 5% and 29% (Iizumi et al., 2014).
Crop management practices maintain or improve yield in function of the environmental offer through the selection of sowing dates based on the varietal requirements. With this strategy, it is possible to escape abiotic stress during critical growth phases or to ensure maximum solar radiation uptake by the crop (Van Ittersum et al., 2008; Zhu et al., 2013). Studies have been carried out around the world to identify optimal sowing dates for different rice varieties to maximize their yield (Akbar et al., 2010; Khalifa et al., 2014; Osman et al., 2015; Pal et al., 2017). However, in Colombia, there are only two evaluation reports that include this practice (Garcés and Restrepo, 2015; Quevedo et al., 2019). Nonetheless, these were evaluated with other varieties that are no longer available for producers and in localities with different edaphoclimatic characteristics; moreover, the impact of these practices was not evaluated in economic terms.
Currently, the increase in crop productivity is due to an increase in fertilization rates. However, in the context of climate change and with limited resources, the indiscriminate application of fertilizers will be a limiting factor in production (Han et al., 2018). In rice crops, nitrogen fertilization is carried out with high doses generating a low efficiency in the use of nitrogen and further pollution problems (Tilman, 2001; Ju et al., 2015). Regarding phosphorus, this element is not available for the plant because it is easily fixed, i.e., through adsorption or precipitation (Roberts and Johnston, 2015). Considering the above and that the majority of the agricultural soils worldwide are deficient in phosphorus (Velázquez et al., 2017), producers usually apply high amounts of this element, generating eutrophication, contamination with heavy metals, and depletion of the resource (Krüger and Adam, 2017).
Based on this problem, several practices have been developed using biological nitrogen fixation processes to increase the availability of the element for the plant (Reinhold and Hurek, 2011). These practices are implemented in addition to the use of phosphorus solubilizing bacteria that transforms the insoluble phosphorus into assimilable forms for the plants (Lavakush et al., 2014). Some of these microorganisms act as plant growth promoters by releasing phytohormones such as auxins, gibberellins, and cytokinins (Castanheira et al, 2014; Shabanamol et al, 2018). This practice is considered eco-friendly and effective to reduce crop fertilization costs (Hallmann et al., 1997), and has also been evaluated with promising results because it increases yield and reduces the fertilization with phosphorus up to 40% (Roger and Ladha, 1992; Lavakush et al., 2014). The use of Azospirillum brasilense and Pseudomonas fluorescens in rice crops increase aerial biomass production, harvest index, and grain yield. This behavior was attribuited to their performance as plant growth promoters (García de Salamone et al., 2012). In Colombia, some products act as nitrogen fixers and phosphorus solubilizers in rice crops. However, there is only one report in Colombia of the effect of Azotobacter chroococcum Beijerinck acting as nitrogen fixating bacteria and plant growth promoter on cotton crops (Romero et al., 2017). However, the effect of this practice at the agronomic and physiological level is not known for rice crops in addition to the financial benefits with its application. Therefore, it was necessary to evaluate some of the products used by rice producers in the country such as the biofertilizer Monibac® based on the nitrogen fixating bacteria A. chroococcum and a biofertilizer under development based on Rhizobium pusense, which operates as a phosphorus solubilizer and plant growth promoter.
Considering the previously mentioned state of the art and that currently there is no knowledge of the effect of the integration of practices, such as the selection of sowing date and biofertilization on rice crops, at the agronomic, physiological and financial levels, our hypothesis is as follows: the selection of the optimal sowing date with the implementation of a biofertilization practice increases the yield and profitability of rice crop. Accordingly, the aim of this study was to evaluate the agronomic, physiological, and financial behavior of variety Fedearroz 68 at three sowing dates with and without biofertilization.
Materials and methods
Study site and plant material
This research was carried out in the municipality of El Espinal, located in the center of the province of Tolima, Colombia, during the year 2017. The experimental site was the Nataima research center of AGROSAVIA georeferenced with coordinates 4°11'28.7" N and 74°57'39.2" W. The soil of the area has a clay-loam texture and is of an alluvial origin. It belongs to the order inceptisol.
The plant material used was the variety Fedearroz 68 that was selected because it is one of the most cultivated varieties in this agro-ecological zone and for its high yield and precocity.
The climatic variables were monitored with a Davis Vantage Pro 2 meteorological station (Davis Instruments, San Francisco, USA).
Experimental design
The study employed a randomized complete block design in a split-plot arrangement. The main plot corresponded to the three sowing dates (SD): May 26, July 7, and August 8, 2017. The subplot corresponded to two mineral nutrition treatments (biofertilization and local production practice). Each treatment was comprised of the SD x nutrition treatment interaction with three replicates, each with an area of 1600 m2.
Application of treatments
Two mineral nutrition treatments were assessed in this study. The first was a local production practice that included the application of chemically synthetized fertilizers in the following rates usually applied by the local producers: 120 kg ha-1 of nitrogen, 80 kg ha-1 of P2O5, and 120 kg ha-1 of K2O. The second is the biofertilization treatment that included the 100% chemical synthesis fertilization applied by the local producers, plus the inoculation with nitrogen-fixing bacteria (A. chroococcum strain AC1 and AC10) at a concentration 1x9 colony forming units (CFU) cm-3, whose commercial name is Monibac® with a dose of1000 cm3 ha-1. Additionally, phosphorus and zinc solubilizing bacteria (R. pusense strain B02) obtained from the germplasm bank of AGROSAVIA microorganisms at a concentration of 1x10 CFU cm-3. These microorganisms were applied at a dose of 500 g ha-1. The bacteria was sprayed to the soil between 21 and 23 d after plant emergence.
Agronomic management of the crop
Mechanized planting was carried out in furrows with a sowing rate of 120 kg ha-1. Additionally, mineral nutrition was divided into four applications administered in the same quantities for both treatments. Agronomic crop management was used at the scale of a commercial crop. Tillage of primary and secondary soil was performed. A mechanized planting by furrows was carried out. Control of weeds using pre-emergent and post-emergent herbicides selective to the crop was also performed: pendimethalin 2500 cm3 ha-1, clomazone 1200 cm3 ha-1, buthaclor 3000 cm3 ha-1, sodium bispiribac 150 cm3 ha-1, cyhalofop 1500 cm3 ha-1. Pest and disease controls were carried out with chemical synthesis products based on periodic monitoring. At phenological stages 30 and 61 the following active ingredients were applied: lambdacihalotrina + thiamethoxam (insecticide) at a dose of 125 cm3 ha-1 and azoxystrobin + diphenoconazole (fungicide) at a dose of 500 cm3 ha-1.
Estimation of gas exchange variables
Net photosynthesis was estimated at phenological stage 65 (i.e., complete flowering: anthers visible in most panicles) and evaluated in completely expanded young leaves using an open system portable gas exchange meter LI-6400 XT (Li-Cor, Lincoln, USA). The photosynthetic photon flux density was 1600 µmol photons m-2 s-1, the concentration of CO2 inside the chamber was 400 µmol CO2 mol-1, and the vapor pressure deficit remained between 1.5 and 1.7 kPa. Data were collected after reaching the steady-state equilibrium (~10 min). Further, the leaf area inside the chamber was measured to correct the data (Quevedo et al, 2019). For the evaluation of respiration and leaf carbon balance (LCB), the methodologies described by Da Silva et al. (2017) were followed, using an open system portable gas exchange meter LI-6400 XT (Li-Cor, Lincoln, USA). The photosynthetic photon flux density was 0 µmol photons m-2 s-1, with a concentration of CO2 inside the chamber of 400 µmol CO2 mol-1. The plants were previously adapted to darkness for 30 min, and the temperature was adjusted inside the chamber with the maximum night temperature of the previous night to simulate field conditions. With the net photosynthesis and respiration data, Equation 1 was used to calculate LCB.
Leaf area index (LAI)
From phenological stages 21 (i.e., start of tillering: first visible tillers) up to 99 (i.e., harvest), the leaf area index (LAI) was estimated every 10 d. To perform such task, a ceptometer ACCUPAR LP-80 (Decagon devices, Hopkins, USA) was used. The evaluation was conducted between 11:00 and 13:00 h. The ceptometer was calibrated according to the manufacturer's instructions, and a leaf distribution value of 0.96 was established. The sensor was located at approximately 45 degrees from the direction of the furrow (Fang et al., 2014).
Interception of photosynthetically active radiation (IPAR), radiation use efficiency (RUE) and harvest index
With the LAI data, third-order logistic regressions were performed, and the daily LAI value was calculated by replacing data in this regression equation. With daily accumulated solar radiation data, daily photosynthetically active radiation (PAR) was estimated, which is equivalent to 50% of the global solar radiation (Garcés and Restrepo, 2015). With this data, Equation 2 was applied to calculate the daily intercepted photosynthetically active radiation (IPAR). The extinction coefficient (k) was assumed to be 0.55, and the calculation of the total IPAR of the crop was carried out by adding the daily IPAR.
Further, Equation 3 was used to calculate the radiation use efficiency (RUE) using the sum of the total solar radiation and the total dry weight obtained at phenological stage 99, as follows:
The harvest index was estimated at phenological stage 99 and the aereal dry matter and the grain weigth were also quantified. Once this information was recorded, the harvest index was calculated (Zhang et al., 2013).
Concentration of nutrients in tissue
One hundred g of foliar tissue were collected from the two youngest leaves during phenological stage 65. These samples were analyzed by spectrophotometry in the Soil and Water Laboratory of the Tibaitata research center (AGROSAVIA), where the nitrogen and phosphorus contents were estimated in percentage, in addition to zinc in mg kg-1.
Yield
At phenological stage 99, four samples of 1 m2 were collected per experimental unit (Garcés and Restrepo, 2015). From these samples, the whole grains were weighed and the green paddy yield was calculated in kg ha-1, and adjusted to a grain moisture content of 22% (Quevedo et al., 2019).
Financial analysis
Financial analysis exercises of SD and nutrition practices as well as their interaction were carried out. In this analysis, cost structures were elaborated where the direct and indirect costs were included. The green paddy rice sale price in US dollars was set at US$0.960 kg-1. With this information, the total utility, profitability, monthly profitability, unit production cost, and unit utility were calculated.
Data analysis
Data analysis was performed using general and mixed linear models considering SD and nutrition as a fixed effect, and the block as a random factor. These models were selected according to the Akaike and Bayesian information criteria. The mean comparison test used was Di Rienzo, Guzmán and Casanoves (DGC). The results show that only the factor or interaction had statistical significance (P≤0.05). Linear and quadratic regression analyses were also performed among the variables LCB, nitrogen content, and yield. The selection of these variables that are highly related to yield was performed using Pearson's correlation coefficient and its P-value. The analyzes described were carried out with the software RStudio version 3.5.1 (RStudio Inc, Boston, USA).
Results
Environmental offer
Table 1 shows the diurnal and nocturnal temperature, and accumulted solar radiation conditions to which the rice plants were subjected. It is noteworthy that the maximum night temperature was lower in the August SD (26.18°C) because it was 0.98°C and 1.24°C below the SD conditions of May and July, respectively. The accumulated solar radiation at the fruit development stage (71-79) was higher for the August SD (4,307.08 Cal cm-2/d) than in July (3,877.34 Cal cm-2/d) and May (4,156.56 Cal cm-2/d).
TABLE 1 Temperature conditions between 70 and 100 days after the emergence of the rice plants established on three different sowing dates.
Sowing date | Phenological state* | Days after emergence | Maximum day temperature (°C) | Minimum night temperature (°C) | Maximum night temperature (°C) | Accumulated solar radiation (Cal cm-2/day |
---|---|---|---|---|---|---|
May | 51-59 | 70-80 | 34.56 | 23.33 | 26.79 | 4,210.01 |
May | 61-69 | 81-90 | 35.80 | 23.88 | 28.21 | 4,565.91 |
May | 71-77 | 91-100 | 32.97 | 23.60 | 26.48 | 4,156.56 |
July | 51-59 | 70-80 | 34.28 | 24.47 | 27.43 | 4,510.17 |
July | 61-69 | 81-90 | 34.31 | 24.24 | 27.62 | 3,775.17 |
July | 71-77 | 91-100 | 32.90 | 23.16 | 27.22 | 3,877.34 |
August | 51-59 | 70-80 | 32.40 | 23.08 | 26.49 | 3,928.64 |
August | 61-69 | 81-90 | 33.56 | 23.17 | 26.43 | 4,139.45 |
August | 71-77 | 91-100 | 30.97 | 22.47 | 25.62 | 4,307.08 |
*indicated phenological state according to the BBCH scale (Lancashire et al., 1991). Beginning of panicle emergence: 51; end of panicle emergence: 59; beginning of flowering: 61; end of flowering: 69; watery ripe: 71; late milk: 77.
Crop yield
Crop yield values showed significant differences for SD factors and nutrition treatments; however, the interaction of these factors did not show significant differences. The SD of August showed the highest yield (8,744.43 kg ha-1 ± 259.24) being 10.22% and 33.43% higher than in July and May, respectively (Fig. 1A). Regarding the nutrition treatments, the biofertilization treatment obtained the highest yield (8,251.88 kg ha-1 ± 178.14), which is 18.92% higher compared to the local production treatment (Fig. 1B).
Dry matter accumulation and harvest index
The dry matter accumulation showed significant differences for SD. The effect of nutrition treatments was not significative. The higher dry matter was for the August SD (4051.95 g m-2 ± 168.31) and the lower dry matter was for the May SD (2830.59 g m-2 ± 113.16) (Fig. 2A). The harvest index showed significative diferences among SD. The August (0.52 ± 0.01) and July (0.53 ± 0.01) SD were equal to a significant level, whereas the May SD showed the lowest harvest index (0.47 ± 0.01) (Fig. 2B).
Radiation use efficiency (RUE)
The RUE showed significant differences for SD factors and nutrition treatments, but not for the interaction of these factors. The SD of August showed the highest RUE (1.31 mg MJ-1 ± 0.03), namely, 17.56 and 41.23% higher than the values obtained in July and May, respectively (Fig. 3A). For the nutrition treatments factor, the biofertilization treatment obtained higher RUE values (1.13 mg MJ-1 ± 0.02) which are 14.16% higher than those obtained with the local production practices treatment (Fig. 3B).
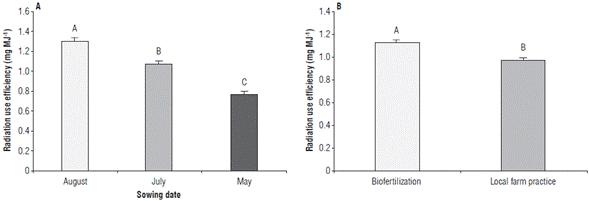
FIGURE 3 Radiation use efficiency in rice plants obtained at three sowing dates with two nutrition treatments. A) radiation use efficiency in three sowing dates, and B) radiation use efficiency for two nutrition treatments. Error bars correspond to the standard error. Different letters indicate treatments with significant differences (P≤0.05).
Leaf carbon balance (LCB)
The LCB showed statistical differences at a significant level only regarding the effect of SD. The SD of August and July are equal at the statistical level (Fig. 4). However, the LCB in the SD of August is 11.56 and 54.04% higher than that of July and May, respectively.
Leaf area index (LAI) and interception of photosynthetically active radiation (IPAR)
The LAI varied significantly only by the SD factor. The SD of August reached a LAI of 10.38, while in May and July it was lower, reaching values of 7.84 and 5.7, respectively; these two SD are statistically equal (Fig. 5). Figure 6 shows the effect of nutrition treatments on the LAI variable. Figures 6A, 6B and 6C show that although the maximum LAI is very similar in each of the SD, with the biofertilization treatment leaf area development is more accelerated compared to the local production practice treatment.
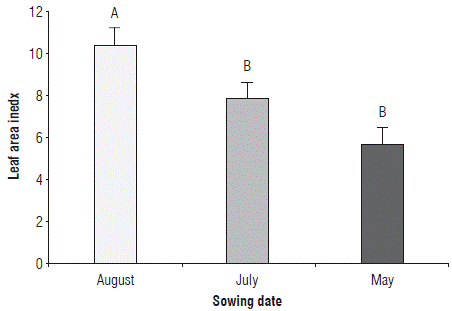
FIGURE 5 Leaf area index of rice plants at phenological stage 65 (i.e., complete flowering: anthers visible in most panicles) at three sowing dates. Error bars correspond to the standard error. Different letters indicate treatments with significant differences (P≤0.05).
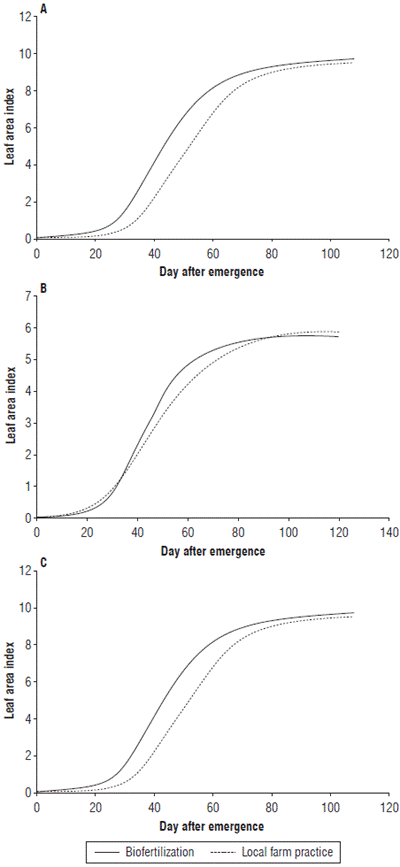
FIGURE 6 Leaf area index for rice plants obtained with two nutrition treatments in three sowing dates (SD). A) May SD, B) July SD, and C) August SD. Error bars correspond to the standard error. Different letters indicate treatments with significant differences (P≤0.05).
Regarding the IPAR, Table 2 shows that this variable was lower for the SD of August compared to the ones of May and July, which showed similar behaviors. Interestingly, the biofertilization treatment in the SD of August increased the IPAR by 10.05%.
TABLE 2 Interception of photosynthetically active solar radiation (IPAR) on three sowing dates under two mineral nutrition treatments.
Sowing date | Nutrition treatment | IPAR (MJ m-2) | Effect of biofertilization (%) |
---|---|---|---|
May | Local farm practice | 732,740.0 | |
May | Biofertilization | 735,393.0 | 0.36 |
July | Local farm practice | 742,940.4 | |
July | Biofertilization | 759,110.4 | 2.18 |
August | Local farm practice | 634,755.5 | |
August | Biofertilization | 698,553.7 | 10.05 |
MJ:Megajoule.
Nutritional status
The nutritional status of the rice plants evaluated by the content of nitrogen, phosphorus, and zinc, only showed significant differences for the SD factor. The effect of nutritional treatments was not significant. The nitrogen content was significantly higher in the SD of August, while the lowest content was observed in May (Fig. 7A). Regarding the phosphorus content, the SD of July and May are equal at a significant level, but higher compared to August (Fig. 7B). As for the zinc content, the SD of May and July showed equal values at a significant level, while in the one of August it was significantly higher compared to the other SD (Fig. 7C).
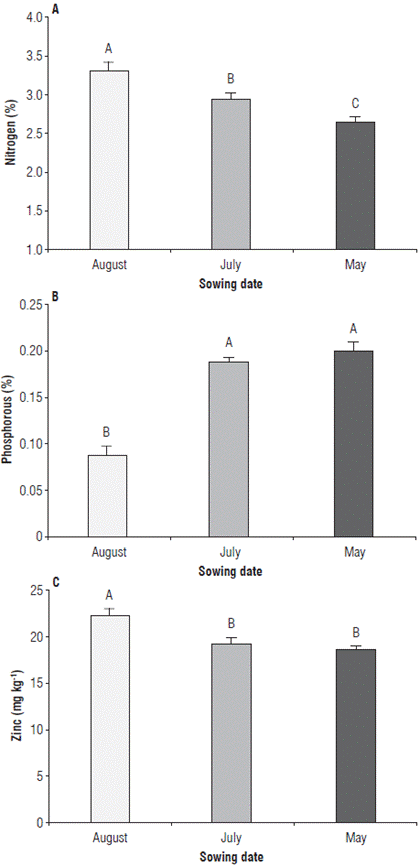
FIGURE 7 Nutritional status of rice plants at phenological stage 65 (i.e., complete flowering: anthers visible in most panicles). A) foliar nitrogen content in rice plants in three sowing dates, B) foliar phosphorus content in rice plants in three sowing dates, and C) foliar zinc content in rice plants in three sowing dates. Error bars correspond to the standard error. Different letters indicate treatments with significant differences (P≤0.05).
Relationship between leaf carbon balance (LCB) and nitrogen concentration with yield
Figure 8 shows the two variables that were most related to yield (LCB and nitrogen concentration). In Figure 8A, a positive linear relationship between LCB and yield is observed. Figure 8B shows a quadratic relationship between nitrogen content and yield, with 3.2% nitrogen at the leaf level observed at the inflection point.
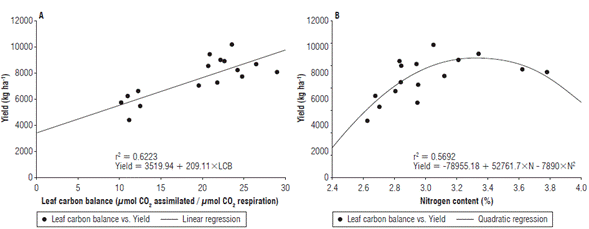
FIGURE 8 Correlation of yield with two variables evaluated at phenological stage 65 (i.e., complete flowering: anthers visible in most panicles). A) correlation of yield with leaf carbon balance, and B) correlation of yield with foliar nitrogen content. Dots correspond to the original data. Solid lines refer to the linear and quadratic regressions.
Financial analysis of the treatments assessed
The financial analysis showed that the economic benefit was affected by the effect of the agronomic management practices (treatments) evaluated. Table 3 shows the financial analysis for the SD x nutrition treatment interaction. This interaction showed that the SD of August with the biofertilization practice showed the highest profit (US$800.73), in which 35.18% profitability was reached; this contrasts with the local production practice that showed 50% lower profitability for this SD. On the other hand, the May SD was highlighted for not obtaining any financial profit, for this reason, negative profitability was found for each of the nutrition treatments, reaching 1.63% and 19.47% for the biofertilization and local production practices, respectively. This allows observing that the exercise of producing rice in this SD is completely unviable in terms of economic efficiency for the evaluated variety (Fedearroz 68). Proof of this is the high production cost per kg of paddy rice produced, which is higher than US$9 for the biofertilization practice, and US$12 for the local production practice compared to the SD of the month of August.
TABLE 3 Financial analysis of the interaction between sowing date and mineral nutrition treatment.
Sowing date | Nutrition treatment | Total sales ($)* | Total costs ($)* | Accumulated profit ($)* | Profitability (%) | Monthly profitability (%) | Unit cost (kg) | Unit profit (kg) |
---|---|---|---|---|---|---|---|---|
May | Biofertilization | 2104.14 | 2139.10 | -34.96 | -1.63 | -0.33 | 0.33 | -0.01 |
May | Local farm practice | 1681.14 | 2087.64 | -406.49 | -19.47 | -3.89 | 0.40 | -0.08 |
July | Biofertilization | 2871.63 | 2388.00 | 483.63 | 20.25 | 4.05 | 0.27 | 0.05 |
July | Local farm practice | 2236.16 | 2313.36 | -77.21 | -3.34 | -0.67 | 0.34 | -0.01 |
August | Biofertilization | 3076.70 | 2275.97 | 800.73 | 35.18 | 7.04 | 0.24 | 0.08 |
August | Local farm practice | 2612.04 | 2219.96 | 392.09 | 17.66 | 3.53 | 0.28 | 0.05 |
*Values are given in United States dollars (USD) with an average exchange rate for 2017.
Additionally, the July SD must be highlighted as it showed a negative return of 3.34% with the local production practice. However, this contrasts with what was obtained with the biofertilization practice that yields a positive return of 20.35%, that is, a net profit of US$483.63. This is mainly due to a lower unit production cost (US$27 kg-1 of rice) which generated US$5 of net profit (Tab. 3).
In the analysis of the SD practice as an independent factor (Tab. 4), the SD in May showed a negative level of profitability (-10.55%) compared to the SD in July and August. Notably, in August, a higher profit of US$1,193 and a 26.42% profitability were obtained.
TABLE 4 Results of the accumulated profit and profitability in three sowing dates under two mineral nutrition treatments.
Sowing date | Profit (US$)* | Profitability (%) | Monthly profit (US$)* | Monthly profitability (%) |
---|---|---|---|---|
May | -441.46 | -10.55 | -88.29 | -2.11 |
July | 406.43 | 8.45 | 81.28 | 1.691 |
August | 1192.81 | 26.42 | 238.56 | 5.28 |
*Values are given in United States dollars (USD) with an average exchange rate of 2017.
Considering the differential result of the yield with the biofertilization practice in the SD evaluated, it was found that it increases a 0.22% in production costs. However, this excess cost allowed achieving 17.93% profitability in the three assessed SD, while the implementation of the local production practice showed a loss of 1.71%. With this exercise, it can be inferred that the implementation of the biofertilization practice allowed achieving US$1,249.4 in profit, while with the local production practice, losses of US$91.62 were obtained in the three SD evaluated, as can be seen in Table 5.
TABLE 5 Accumulated profit and profitability for two mineral nutrition treatments during three sowing dates.
Nutrition | Profit | Profitability | Monthly profit | Monthly |
---|---|---|---|---|
treatment | (US$)* | (%) | (US$)* | profitability (%) |
Biofertilization | 1249.4 | 17.93 | 249.88 | 3.58 |
Local farm practice | -91.62 | -1.71 | -18.324 | -0.34 |
*Values are given in United States dollars (USD) with an average exchange rate of 2017.
Discussion
Rice yield is influenced significantly by the effect of the SD. The highest yield was obtained in the August SD, so the environmental offer in this SD is optimal for the Fedearroz 68 variety. Nonetheless, high RUE values were observed, and this variable is related to the amount of solar radiation, the capacity of the canopy to capture solar radiation and the efficiency of its conversion into phytomass (Parry et al., 2011; Ceotto et al., 2013). In this case, the accumulated solar radiation at the late milk stage in the August SD (4,307.08 Cal cm-2/day) was higher than other SD. The solar radiation collection capacity estimated by the LAI was higher than 10 during the August SD, which is considered the optimal LAI for rice plants to obtain a maximum yield (Mae et al., 2006; Quevedo et al., 2019). However, it is noteworthy that the lowest IPAR was obtained during this SD, which is contradictory to what was found by Garcés and Restrepo (2015) in the municipality of Saldaña (province of Tolima), where they identified that the SD where the highest IPAR value was obtained was the one with the maximum yield (7000 kg ha-1). This may be because the Fedearroz 68 variety in the presence of high radiation suffers a photoinhibition phenomenon.
For the efficiency in the conversion of solar radiation into carbohydrates, the LCB was directly related to the yield. For this reason, in the SD of August, the highest yield and the LCB reached 25 µmol CO2 / µmol CO2 respiration; this, however, is explained by the lower nighttime temperature that occurred in this SD. The above is favorable for rice plants since this environmental condition decreases their respiration rate (Alvarado et al., 2017). Furthermore, this condition affects the availability of carbohydrates for grain filling, so the magnitude of respiration can increase or reduce yield (Peraudeau et al., 2015). The high night temperature could generate damage of cell membrane by the generation of reactive species of oxygen (Xue et al., 2012). The partitioning to developing sink (grain) was determinated through the harvest index which is affected by the high night temperature (Zhang et al., 2013). In this research, it was observed that the harvest index and dry matter accumulation were higher on the August and July SD, when the night temperature was lower than in the May SD. This is related with the high yield on the August SD and the low yield on the May SD.
Regarding the ability to fix CO2, no clear relationship was found between the net photosynthesis evaluated at the leaf level and crop yield, which is contradictory to what Hidayati et al. (2016) found. However, it is hypothesized that in the SD of August the photosynthesis at the canopy level was higher than in the other SD, given that only in this SD a nitrogen concentration higher than 3% was reached, which is considered ideal (Ray, 2013). Nonetheless, this can be related to a high concentration of chlorophyll (Lee et al., 2011) and RuBisCO (Imai et al., 2008). In addition to this, authors as Mae et al. (2006) consider that the optimal LAI has a value of 10.38, so considering the above mentioned, the photosynthesis at the canopy level may be higher compared to the other SD. However, this hypothesis must be verified in future studies.
The nitrogen content was related to yield in a quadratic way; hence, the maximum yield was found with a foliar nitrogen content of 3.3%. However, if the content is higher than this value, the yield shows a decreasing tendency. This can be attributed to the higher availability of the element in the soil solution, which increases vegetative development, and specifically, the development of late tillers that affect the IPAR and the distribution of carbohydrates (Wang et al., 2017).
Regarding the effect of the nutrition treatments on yield, biofertilization was found to increase yield by 18.92%; this was similar to what was found in other studies in which this practice increased yield between 19.8% and 29.32% (Roger and Ladha, 1992; Lavakush et al., 2014). However, no significant changes were observed in the concentration of nitrogen, phosphorus, and zinc in tissue at phenological stage 65 as a result of this practice. This suggested that the biofertilization did not increase the availability in soil of nitrogen, phosphorus and zinc. Despite this, biofertilization affected the growth level because the plants under this treatment developed their LAI more quickly, which allowed increasing IPAR up to 10.05%. This behavior can be attributed to the fact that the phosphate solubilizing bacterium R. pusense strain B02 and A. chroococcum act as plant growth promoters as they produce indole acetic acid that is an auxin (Castanheira et al., 2014; Romero et al., 2017) controlling cell division and foliar expansion processes (Li et al., 2007). Moreover, they have also been found to affect the degree of foliar inclination, which generates a higher leaf area exposed to light (Zhao et al., 2013). For these reasons, it is believed that leaf development and IPAR were higher with the biofertilization treatment. By increasing the uptake of light, the CO2 fixation capacity increases (Garcés and Restrepo, 2015), so the plant would have greater availability of photoassimilates to fill a higher number of demanding organs, producing the increase in yield that was observed in this study.
The financial analysis of the evaluated practices allowed estanblishing the level of productivity of the local production practice in economic terms. In this practice, low percentages of profitability were obtained, which in many cases generates economic losses. The above was evidenced in two of the three SD evaluated; the SD of May showed a negative return of 10.55% with losses of US$441.4, while in the SD of July there was a positive profitability of 8.45% and a profit of US$406.46. The latter differs with the results found by Akbar et al. (2010), who evaluated the Super Basmati variety in six SD, estimating a loss of US$40.47 ha-1. Additionally, Bashir et al. (2010) also reported that the July SD cultivated with the KS-282 variety recorded a loss of US$184.54. In contrast, Osman et al. (2015) stated that this SD shows a higher net profit with US$746.07 ha-1 in the semi-arid conditions of Sudan, which reach a yield of 2900 kg ha-1 with the Nerica 4 variety. A 17.66% profitability was obtained in the August SD with the local production practice, However, with the implementation of the biofertilization practice, the profitability increased up to 35.18%. This practice was also crucial in the July SD, given that it showed a return of 20.25% yielding a cumulative profit of US$1,284.36 ha-1, while with the local production practice, a negative return of -3.34% was obtained. Considering the above, we can conclude that the use of biofertilizers is a viable alternative from the technical and economic points of view, which is similar to what was stated by Mohammadi and Sohrabi (2012), who also reported that the use of biofertilizers plays a key role in soil productivity and sustainability.
Conclusions
Crop yield and profitability was affected by the management practices evaluated. With the selection of the sowing date, it was possible to maximize the yield of the Fedearroz 68 variety, given by the occurrence of low nocturnal temperatures during flowering and an ideal range of solar radiation. With the biofertilization practice, plant growth was improved allowing a better light interception and, therefore, higher radiation use efficiency.
The selection of the sowing date does not change the production costs but increases the profitability of up to 26.42%. Further, with the biofertilization practice, the production cost increases by 0.22%, while the profitability increases by up to 35.18%. This allowed reducing the production unit cost to US$0.24 kg-1. For this reason, we conclude that these practices are viable from the technical and economic points of view because they increase productivity and economic benefit. However, this should be evaluated in other varieties and agroecological zones, to allow the elaboration of recommendations for a large part of the rice producing areas of Colombia.