Services on Demand
Journal
Article
Indicators
-
Cited by SciELO
-
Access statistics
Related links
-
Cited by Google
-
Similars in SciELO
-
Similars in Google
Share
Ciencia y Tecnología Agropecuaria
Print version ISSN 0122-8706On-line version ISSN 2500-5308
Cienc. Tecnol. Agropecuaria vol.20 no.2 Mosquera May/Aug. 2019
https://doi.org/10.21930/rcta.vol20num2art:1458
Alimentación y nutrición animal
Bromatological and structural changes in Megathyrsus maximus in four silvopastoral arrangement systems
1Investigador máster, Corporación Colombiana de Investigación Agropecuaria (Agrosavia), ci Turipaná. Cereté, Colombia.
2Investigadora PhD (en retiro), Corporación Colombiana de Investigación Agropecuaria (Agrosavia), ci Turipaná.Cereté, Colombia.
3Corporación Colombiana de Investigación Agropecuaria - Corpoica
This study aimed to compare the bromatological and structural behavior of Megathyrsus maximus (Poaceae) in four silvopastoral arrangement systems and a treeless meadow. Exclusion cages were used to evaluate four regrowth ages: 7, 14, 21 and 28 days. In each stage, the following variables were assessed: crude protein, neutral detergent fiber, acid detergent fiber and in situ dry matter digestibility (bromatological), as well as plant height, leaf: stem ratio and leaf width (structural). Crude protein in M. maximus was affected by the silvopastoral arrangement (p < 0.05). The crude protein in the Pasto and p-Arbust treatments were 2.42 % lower compared to the means of the treatments p-Arbor, p-Arbust-Arbor, and p-Arbust-Arbor-M. The neutral (65.16 %) and acid (37.30 %) detergent fiber contents were higher in the dry season (p < 0.05). Regarding the structural response, treatment affected (p < 0.05) plant height, leaf: stem ratio and leaf width, with the highest values shown by p-Arbor (80.26 cm), Pasto (51.75:1 g), and p-Arbust-Arbor (2.73 cm), respectively. Interaction among age, season and arrangement was recorded (p < 0.05) in leaf height and width. In the silvopastoral arrangement system with tree cover, leaf height and width were higher at 28 regrowth days in the dry season, compared with the systems without trees. In conclusion, the silvopastoral arrangement system influenced the adaptative response of M. maximus, with an increase in crude protein and better response in leaf height and width.
Keywords Megathyrsus maximum; Mombasa; nutritive value; Panicum maximum; phenotypic plasticity; silvopastoral systems
El objetivo de este estudio fue comparar el comportamiento bromatológico y estructural de Megathyrsus maximus (Poaceae) bajo cuatro arreglos silvopastoriles y una pradera sin árboles. Se utilizaron jaulas de exclusión para evaluar cuatro edades de rebrote: 7, 14, 21 y 28 días. En cada edad, se registró la proteína cruda, fibra en detergente neutro, fibra en detergente ácido y digestibilidad in situ de la materia seca (bromatológico), así como altura de planta, relación hoja:tallo y ancho de la hoja (estructural). El arreglo silvopastoril afectó el contenido de proteína en M. maximus (p < 0,05). El promedio de la proteína en los tratamientos Pasto y p-Arbust fue 2,42% menor que el promedio de los tratamientos p-Arbor, p-Arbust-Arbor y p-Arbust-Arbor-M. Los contenidos de fibra en detergente neutro (65,16 %) y ácido (37,30 %) fueron mayores en época seca (p<0,05). En la respuesta estructural, el tratamiento afectó la altura, la relación hoja:tallo y ancho de la hoja (p< 0,05), con los mayores registros en p-Arbor (80,26 cm), Pasto (51,75:1 g) y p-Arbust-Arbor (2,73 cm), respectivamente. Se registró interacción de la edad, la época y el arreglo (p< 0,05) en la altura y ancho de la hoja. En estas características, los arreglos silvopastoriles con cobertura arbórea evidenciaron una mejor respuesta en época seca a 28 días, comparado con los sistemas sin árboles. Se concluye que el arreglo silvopastoril influyó en la respuesta adaptativa de M. maximus, con incremento de proteína y mejor respuesta en alto y ancho de hoja.
Palabras clave Megathyrsus maximum; Mombasa; Panicum maximum; plasticidad fenotípica; sistemas agrosilvopastoriles; valor nutritivo
Introduction
The silvopastoral systems (sps) are characterized as agroforestry arrangements that include shrubs, trees or timber species together with a herbaceous component generally associated with grasses (Pezo & Ibrahim, 1998; Sinclair, 1999), which can range from intensive (> 10,000 shrubs/ha) without widespread tree cover (Calle et al., 2013), up to multistratified with low density (< 100 trees/ha) and widespread tree cover (Cajas-Giron & Sinclair, 2001).
In the case of multi-stratified systems, the facilitating interactions (reduction of evapotranspiration, nitrogen fixation, among others) and competition (water, light and nutrients) between the arboreal and the herbaceous components are essential elements in the sustainability and productivity of the system (Dohn et al., 2013; Guenni, Seiter, & Figueroa, 2008; Martínez, Cajas, León, & Osorio, 2014), especially under grazing conditions. However, sometimes the potential for herbaceous productivity in SPS with tree cover becomes limited by the shadow effect (Abraham et al., 2014; Paciullo et al., 2017). Usually, intercepts of incident radiation above 50 % may affect the production of biomass in tropical grasses (Guenni et al., 2008; Paciullo et al., 2017; Villanueva, Ibrahim, & Ríos, 2008), due to the reduction in the photosynthetic rate, especially in C4 species (Dohn et al., 2013; Santiago-Hernández et al., 2016).
The interactions between ca nopy cover and the productivity of herbaceous species are not entirely linear, particularly in grass species that may exhibit morphological and adaptive plasticity attributes (Abraham et al., 2014; Guenni et al., 2008). This term has been described as the ability of some plants to change biochemical, morphological and physiological attributes, in response to temporary or permanent environmental variations (De Kroon, Huber, Stuefer, & Van Groenendael, 2005; Dias-Filho, 2002). According to Guenni et al. (2008), these adaptations are mostly associated with respiratory rate reductions, increases in the aerial/root component relations, and increases in the specific leaf area.
In this sense, other authors have also cited leaf stretching (Paciullo et al., 2017), the reduction in the appearance rate of stems, and increases in their length (Abraham et al., 2014).
In grasses (Poaceae) of the genus Megathyrsus, evidence of adaptive plasticity has been reported under tree shade conditions, with an increase in morphological attributes such as plant height, leaf width, length and stem appearance rates (Medinilla-Salinas et al., 2013; Paciullo et al., 2017; Santiago-Hernández et al., 2016; Silveira, Dos Santos, Rodrigues, Rodrigues, & Alencar, 2017; Viafara, Clavero, & Araujo-Febres, 1997), as well as biochemical attributes such as increases in crude protein content (Durr & Rangel, 2003; Paciullo et al., 2017; Santiago-Hernández et al., 2016).
The behavior of the herbaceous component under tree cover conditions in a SPS is a fundamental part of its success and adoption. However, there is still little knowledge about the potential of grasses in sps with tree cover and light restriction (Silveira et al., 2017).
The current study aimed to compare the bromatological and structural behavior of Megathyrsus maximus ( Jacq.) B.K. Simon & S.W.L. Jacobs (Poaceae), under four multi-strata silvopastoral arrangements and a meadow without trees.
Materials and methods
Study area
The evaluation was carried out at the Turipaná research center linked to Corporación Colombiana de Investigación Agropecuaria (AGROSAVIA), located in Cereté, Córdoba, Colombia (08°51' N, 75°49' W; 18 m.a.s.l.). The area is located in the Caribbean region and has two defined climatic periods: a rainy season that occurs from May to November and a dry one from December to April. The average annual rainfall is 1,380 mm, with an average annual temperature of 28 °C. During the year, potential evapotranspiration of 1,240 mm and a relative humidity of 81 % were recorded (data from the AGROSAVIA-Turipaná climatic station). According to the life zones classification of Holdridge (1971), the area is categorized ecologically as tropical dry forest (bs-T).
In 1998, four types of silvopastoral systems of different structure and complexity, as well as a treeless meadow (control) were established (Cajas-Giron & Sinclair, 2001). The silvopastoral arrangements consisted of three different strata: pastures, shrubs, and trees (for fruit or timber production). The control treatment (meadow) consisted only of pastures (Cynodon nlemfuensis Vanderyst, Dichanthium aristatum (Poir.) C. E. Hubb., and Megathyrsus maximus). The first silvopastoral arrangement (p-Arbust) included only shrubs: Leucaena leucocephala (Lam.) De Wit. and Crescentia cujete L.; the second included a stratum of trees (p-Arbor): Cassia grandis L. F., Pithecellobium saman ( Jacq.) Benth., and Guazuma ulmifolia Lam.; the third had a combination of pastures, shrubs, and trees (p-Arbust-Arbor); and the fourth arrangement (p-Arbust-Arbor-Mad) included the previous strata plus the inclusion of timber species: Pachira quinata W.S. Alverson and Swietenia macrophylla King (table 1).
The experiment was carried out in an area of 30 ha divided by a differential drainage effect in three sectors characterized as blocks (poor, moderate and optimal drainage). In each block, a replicate of the evaluated treatments was established in an area of 2 ha (100 m wide by 200 m long), for a total of three replicates per treatment and 15 plots (figure 1). Each treatment was divided into five strips of 4,000 m2 subject to a grazing scheme of 2 days of occupation and 28 days of rest.
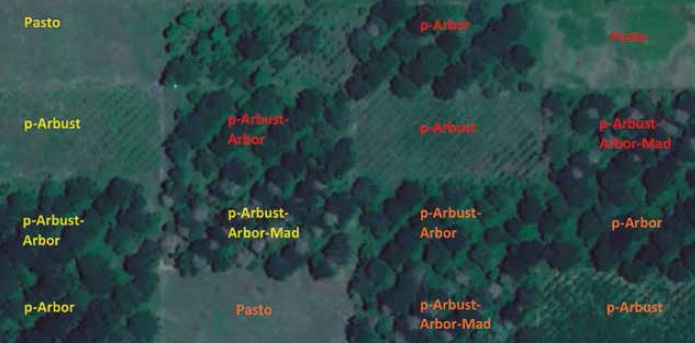
Source: Elaborated by the authors employing a satellite image of DigitalGlobe® Google 2019. Photograph at 1,338 m abovethe terrain.
Figure 1. Satellite image of the spatial distribution of the silvopastoral systems assessed in CI Turipaná (agrosavia), Cereté (Córdoba, Colombia). Block 1: orange; block 2: red, and block 3: yellow.
Data recording
The bromatological and structural changes occurred in M. maximus were evaluated at four regrowth ages: 7, 14, 21 and 28 days. In each treatment, four central points were selected to avoid the edge effect and to receive the shade conditions of each silvopastoral arrangement system. At each point (after cutting the grass at 25 cm from the ground to standardize plant height) an exclusion cage was installed (2 m long x 0,5 m wide; area of 1 m2), built in mesh and metal armor to avoid foraging of grazing animals. Each cage represented a cutting age.
In each of the plots, the following variables were measured. Plant height (in centimeters), registering the average value of the five highest flag leaves (Viafara et al., 1997; Wong & Wilson, 1980). Leaf width (in centimeters) from the broadest middle part of the leaf, registering the average value of 5 leaves in the plot (Guerin, Wen, & Lowe, 2012). Leaf: stem (lsr) ratio, obtained from the total harvest of the plot and manual separation of leaves and stems, that were subsequently dried in a forced ventilation oven at 60 °C for 48 hours. The ratio was calculated by dividing the dry weight of the leaves by the dry weight of the stems.
In each age, a forage subsample was harvested for bromatological analysis. The forage samples were analyzed in the Animal Nutrition Laboratory of agrosavia, ci Turipaná, where the crude protein (cp), the neutral detergent fiber (ndf) and the acid detergent fiber (adf) were measured according to the methods of the Association of Official Analytical Chemists (AOAC, 2002). Further, in situ dry matter digestibility (isdmd) according to the nylon bag technique described by Ørskov, Hovell and Mold (1980) was measured. Information recording was carried out during the rainy season (August-November 2010) and also during the dry season ( January-April 2011), in which two repetitions were carried out each.
Statistical analysis
The information was collected under a completely randomized block design structure. Each of the records was registered in spreadsheets for review and subsequent analysis. Data analysis was carried out using a mixed model according to repeated measures. For each variable, the random effects were modeled by an over-adjustment of the fixed part of the model (season, treatment and age). After the identification of the best random effects structure (constant variance of the block plus the random variance for each of the ages minus one factor levels), the fixed part was modeled and evaluated from the model that did not include interactions (simplest model) up to the model that had all the possible interactions, using a maximum-likelihood estimator. After the identification of the best structure describing both the fixed and the random parts through the aic and bic information criteria (data not shown), an analysis of the variables was carried out using the restricted maximum-likelihood estimator (Cayuela, 2018; Di Rienzo, Macchiavelli, & Casanoves, 2009). Data analysis was performed with the lme function of the nlme statistical package (Pinheiro, Bates, DebRoy, & Sarkar, 2018) in the R-Project software (Team R, 2018). In the case of the variables adf, plant height, lsr and leaf width that did not fulfill the assumptions of normality and homogeneity of variance, these were transformed using the logarithm function. When the null hypothesis was rejected, the Tukey test was used to perform the separation of the means. In all cases, 0.05 was considered as a critical value.
Results and discussion
The treatment significantly affected (p < 0.05) the proportion of CP in M. maximus. The average CP in the Pasto and p-Arbust treatments (without tree coverage) was 2.42 percentage points lower compared to the average values found in the p-Arbor, p-Arbust-Arbor and p-Arbust-Arbor-M treatments (with tree coverage). In the case of the fibers, an effect (1 < 0.05) of the season was observed on the neutral and acid detergent fibers. The ndf and the adf for the dry period were higher (65.16 % and 37.30 %, respectively) compared to the values registered in the rainy period (63.28 % and 37.30 %, respectively). As expected, age significantly affected all the variables evaluated (p < 0.05), with an increase for fibers and a decrease in protein and in situ dry matter digestibility, from 7 to 28 days (table 2).
Table 2. Bromatological composition in percentages of Megathyrsus maximus in four silvopastoral arrangements and a meadow without trees, in CI Turipaná during 2011 cp: Crude protein; ndf: Neutral detergent fiber; adf: Acid detergent fiber; isdmd: In situ dry matter digestibility; sem:Standard error of the mean.
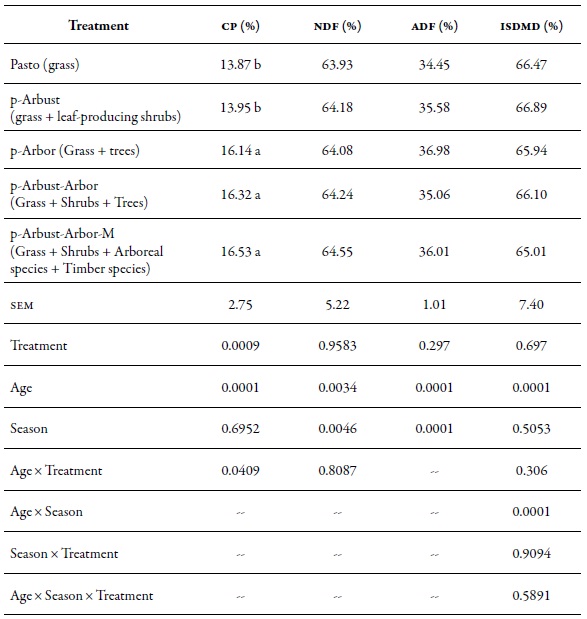
Source: Elaborated by the authors
Note:Different letters between averages in the same column indicate significant statistical difference at p < 0.05, according tothe Tukey test.
The interaction effect of the treatment and age was observed (p < 0.05) for the CP variable. All the treatments show a decreasing tendency for cp content as age advances. However, the trend is more accentuated in the Pasto and p-Arbust treatments (without tree cover), compared with the p-Arbor, p-Arbust-Arbor and p-Arbust-Arbor-M treatments (with tree cover). In day 14, the results obtained for CP differs significantly between the group of treatments with and without tree coverage (figure 2).
Table 3 illustrates the structural behavior of M. maximus evaluated according to four silvopastoral arrangements and the treeless meadow. The treatment significantly affected (p < 0.05) plant height, LSR and leaf width. The p-Arbor treatment showed the highest average height per plant (80.2 cm), which significantly exceeded (p < 0.05) the average height recorded in the Pasto treatment (68.8 cm). This behavior was consistent with the leaf width variable, finding the broadest leaf values in the p-Arbor and p-Arbust-Arbor treatments (2.68 cm and 2.71 cm, respectively) with a significant difference compared to the Pasto treatment (2.16 cm). The LSR variable showed a contrary trend to the variables described above. The Pasto treatment registered the best leaf: stem ratio with 51.7 g of leaves per gram of stem. This record was significantly different (p < 0.05) from the records reported in the treatments with arboreal coverage, i.e., p-Arbor (16.6 g), p-Arbust-Arbor (13.6 g) and p-Arbust-Arbor- M (16.5 g).
Nonetheless, the season significantly affected (p < 0.05) leaf height and width. In the dry season the highest plant height (79.89 cm) and, on average, the widest leaf (2.61 cm) was obtained, compared to the rainy period (70.19 cm and 2.41 cm, respectively).
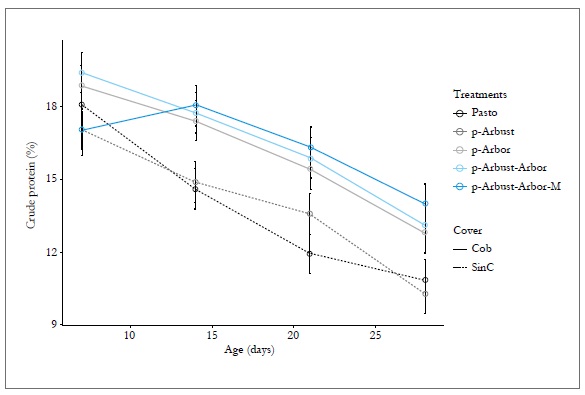
Source: Elaborated by the authors
Figure 2. Effect of the silvopastoral arrangement and age on the crude protein content of Megathyrsus maximus, in ci Turipaná during 2011. Cob = Tree cover; SinC = No tree cover. Vertical bars indicate the standard error of the mean.
Table 3. Structural characteristics of Megathyrsus maximus in four silvopastoral arrangements and a meadow without trees, in CI Turipaná during 2011 lsr: Leaf: stem ratio; sem: Standard error of the mean.
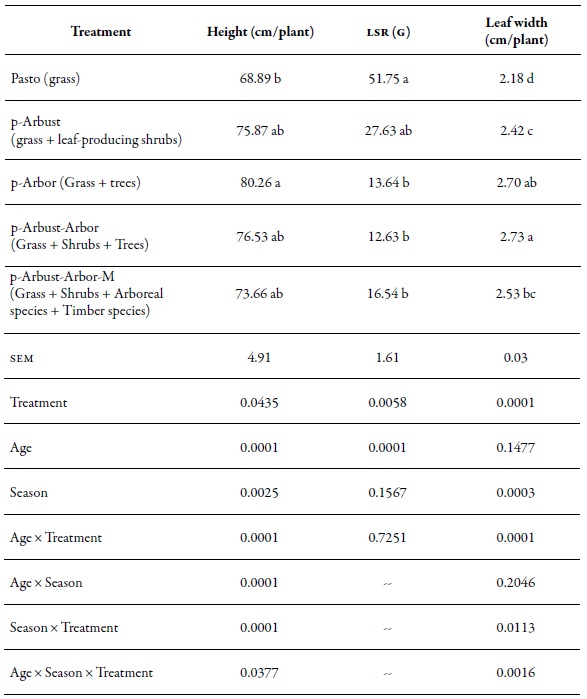
Source: Elaborated by the authors
Note:Different letters between averages in the same column indicate significant statistical difference at p < 0.05 according tothe Tukey test.
The interaction of the SPS arrangement, the age and the season showed a significant effect (p < 0.05) on plant height and leaf width. The height for the dry period at 28 days was higher in the SPS arrangements p-Arbor (104.77 cm), p-Arbust-Arbor (102.62 cm) and p-ArbustArbor-M (111.31 cm), compared with treatments without tree cover (86.39 cm in p-Arbust and 70.97 cm in Pasto). Further, leaf width showed similar behavior to plant height. During the dry period after 28 days of regrowth, the SPS arrangements with tree cover registered the highest averages leaf width values (2.80, 2.81 and 2.74 cm for p-ArbustArbor-M, p-Arbust-Arbor and p-Arbor, respectively), compared with the Pasto and the p-Arbust treatments, which recorded an average leaf width of 2.1 and 2.3 cm, respectively (figure 3).
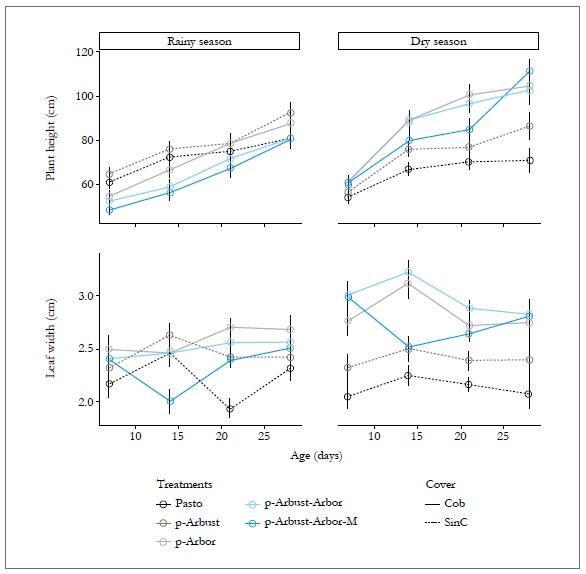
Source: Elaborated by the authors
Figure 3. Effect of the silvopastoral arrangement and the age in some structural characteristics of Megathyrsusmaximus, in ci Turipaná during 2011. Cob = Tree cover, SinC = No tree cover. Vertical bars indicate the standarderror of the mean.
The compositional and morphological changes observed in M. maximus are consistent with the descriptions reported for C4 species under shade (Sage & McKown, 2006). C4 plants, such as M. maximus (Giraldo-Cañas, 2010) have evolved to adapt to highly luminous and hot environments with low CO2 saturation, through biochemical and anatomical modifications that focus on carbon fixation in the RuBisCO enzyme (Edwards & Smith, 2010). However, the plasticity of C4 plants to shade conditions revolves around the carbon economy, in which the plant increases its photosynthetic efficiency by increasing the leaf area, decreasing leaf thickness, by changing the aerial/root proportions and increasing chlorophyll concentration. All these changes are mediated by nitrogen availability (Valadares & Niinemets, 2008), which can be influenced by the presence of leguminous trees (Pentón & Blanco, 1997).
In this work, the bromatological changes of M. maximus under shade were evident, mainly for cp, with a difference of at least 2.4% between the SPS with tree cover and the p-Arbust and Pasto treatments. Authors such as Paciullo et al. (2017) report increases in cp content for M. maximus in 3.3 % under artificial shade conditions (up to 58 % retention with black polypropylene cloth); moreover, Santiago et al. (2016) recorded increases in leaves of 2.5 % in CP and 0.8 % in stems under the coverage of Melia azedarach L.
On the other hand, Durr and Rangel (2003) reported increases of 4.7 % in cp content under the shade of Pithecellobium saman. Contrary to this, when Obispo, Espinoza, Gil, Ovalles and Rodríguez (2008) evaluated shadow levels between 10 % and 30 % generated by P. saman, they did not report a significant effect of the shadow on CP content in M. maximus, like Medinilla et al. (2013) under coverage of Gliricidia sepium ( Jacq.) Kunth ex Walp.; the latter was probably influenced by the decrease in organic carbon contents in the soil during the experimental period.
Regarding the increase of cp in sps, Cruz (1997) has reported that nitrogen increases in the grass under tree shade are associated to phenomena such as the recycling of nutrients through the fall of litter and the facilitation of nitrogen promoted by legumes species. Moreover, Martínez et al. (2014) evaluating the sps arrangements object of the current study reported that under the arrangements with tree coverage (p-Arbor, p-Arbust-Arbor, and p-Arbust-Arbor-M), the nutrient recycling dynamics improve the contents of P, K, Ca and organic matter, compared with the meadow without trees.
Also, authors such as Abule, Smit and Snyman (2005), through the evaluation of the influence of trees on plant composition and soil nutrients, have created the term "fertility island" to describe the improvements of nitrogen and organic matter under tree canopies generated by the interaction between soil microfauna and the accumulation of litter. In this sense, Avendaño-Yanez, López-Ortiz, Perroni and Pérez-Elizalde (2018) established that natural tree systems (Vachellia pennatula (Schltdl. & Cham.) Seigler & Ebinger and Lysiloma acapulcense (Kunth) Benth.), and pastures (M. maximus and Hyparrhenia rufa (Nees) Stapf) increase the contents of organic carbon, organic matter, ammonium, and total phosphorus, through mechanisms such as nitrogen fixation, and nitrogen and phosphorus sequestration by microbial activity, and through litterfall and decomposition.
These increases in protein may also be associated with physiological and biochemical adaptations evidenced in shaded grasses. In this regard, Penton and Blanco (1997) affirm that, under shade conditions, an accumulation of nitrates can occur in the absence of carbohydrates necessary for their reduction to ammonia. This accumulation of nitrates eventually leads to an increase in the percentage share of the protein (N x 6.25) in the dry matter. Similarly, Dias-Filho (2002) indicates that C4 grass species can significantly increase the total concentration of chlorophyll under shade, compared with the same grass under full sun exposure. This increase in chlorophyll is due to the possibility of arranging nitrogen for the production of molecules and proteins linked to photosynthetic pigments (Valadares & Niinemets, 2008), in order to improve efficiency in photosynthesis.
The structural changes evidenced in plant height, leaf width and the LSR values influenced by the silvopastoral arrangements in M. maximus, can be related to the morphological and adaptive plasticity of the grass, and to environmental micro-changes (greater light income) generated by the system when passing from the rainy period to the dry period. In this transition, the trees (for fruit and timber production) experience a total (P. quinata and P. saman) or partial (C. grandis and G. ulmifolia) defoliation phenomena as a defense mechanism against the decrease in rainfall (Olivero-Lora, 2011, Rusch et al., 2014). The higher light income, as a result of the loss of leaves in some fruit and timber trees during the dry period, could stimulate the morphological plasticity of M. maximus, which resulted in a higher increase in the average height of the plant and width of the leaves in the p-Arbor, p-Arbust-Arbor and p-Arbust-Arbor-M arrangements.
The elongation of the stems in grasses under shade conditions is considered an adaptive mechanism that seeks to increase their competitive capacity and improve the distribution of light along the canopy (Guenni et al., 2008); similarly, the width of the leaf reflects an adaptive response of plant species subjected to stress conditions (Baruch et al., 2017; Guerin et al., 2012). Paciullo et al. (2017) recorded stem elongation rates of 0.06 to 0.19 mm/day in M. maximus from 0 to 58 % light interception. Medinilla et al. (2013) reported increases of 12 % in plant height for M. maximus under the shade of G. sepium, compared to growth under full sun exposure. Regarding leaf width, Gómez, Navarro and Pérez (2016) indicated that under the shade of P. saman, M. maximus registered a significant increase of 0.3 cm, compared with the grass under full sun exposure.
Conclusions
Silvopastoral systems with tree cover generated an adaptive response in M. maximus in variables such as crude protein content, plant height, leaf width, and the leaf: stem ratio. Results showed that the grass was adapted to the light reduction conditions in the sps, which is promising in the management of grazing, with possible benefits in the contribution of forage with higher protein quality to the diet of grazing animals.
Disclaimer
This research was developed within the framework of the project "Comprehensive Assessment of the Benefits of Multiple Strata Silvopastoral Systems on Soil Sustainability and Animal Productivity in the Dual Purpose System of the Caribbean Region of Colombia", funded by Ministerio de Agricultura y Desarrollo Rural (madr) [2010 Research Call] and executed by AGROSAVIA (formerly Corpoica).
REFERENCES
Abraham, E. M., Kyriazopoulos, A. P., Parissi, Z. M., Kostopoulou, P., Karatassiou, M., Anjalanidou, K., & Katsouta, C. (2014). Growth, dry matter production, phenotypic plasticity, and nutritive value of three natural populations of Dactylis glomerata L. under various shading treatments. Agroforestry Systems, 88(2), 287-299. doi: 10.1007/s10457-014-9682-9. [ Links ]
Abule, E., Smit, G. N., & Snyman, H. A. (2005). The influence of woody plants and livestock grazing on grass species composition, yield and soil nutrients in the Middle Awash Valley of Ethiopia. Journal of Arid Environments, 60(2), 343-358. doi:10.1016/j.jaridenv.2004.04.006. [ Links ]
Association of Official Analytical Chemist (AOAC). (2002). Official methods of analysis of AOAC international (17th ed.). Gaithersburg, EE. UU.: autor. [ Links ]
Avendaño-Yáñez, M. de la L., López-Ortiz, S., Perroni, Y., & Pérez-Elizalde, S. (2018). Leguminous trees from tropical dry forest generate fertility islands in pastures. Arid Land Research and Management, 32(1), 57-70. doi:10.1080/15 324982.2017.1377782. [ Links ]
Barragán-Hernández, W. A., Mahecha-Ledesma, L., & Cajas-Girón, Y. S. (2015). Variables fisiológicas-metabólicas de estrés calórico en vacas bajo silvopastoreo y pradera sin árboles. Agronomía Mesoamericana, 26(2), 211-223. doi:10.15517/am.v26i2.19277. [ Links ]
Baruch, Z., Christmas, M. J., Breed, M. F., Guerin, G. R., Caddy-Retalic, S., McDonald, J., … Lowe, A. J. (2017). Leaf trait associations with environmental variation in the wide-ranging shrub Dodonaea viscosa subsp. angustissima (Sapindaceae). Austral Ecology, 42(5), 553-561. doi:10. 1111/aec.12474. [ Links ]
Cajas-Giron, Y. S., & Sinclair, F. L. (2001). Characterization of multistrata silvopastoral systems on seasonally dry pastures in the Caribbean Region of Colombia. Agroforestry Systems, 53(2), 215-225. doi:10.1023/A:1013384706085. [ Links ]
Calle, Z., Murgueitio, E., Chará, J., Molina, C. H., Zuluaga, A. F., & Calle, A. (2013). A Strategy for Scaling-Up Intensive Silvopastoral Systems in Colombia. Journal of Sustainable Forestry, 32(7), 677-693. doi:10.1080/10549811.2013.81 7338. [ Links ]
Cayuela, L. (2018). Modelos lineales mixtos (LMM) y modelos lineales generalizados mixtos (GLMM) en R. Recuperado de https://www.dl.orangedox.com/XpAzAQ0duvnoBmWZJ2/5-Modelos%20lineales%20mixtos%20en%20R.pdf [ Links ]
Cruz, P. (1997). Effect of shade on the growth and mineral nutrition of a C 4 perennial grass under field conditions. Plant and Soil, 188(1990), 227-237. [ Links ]
De Kroon, H., Huber, H., Stuefer, J. F., & Van Groenendael, J. M. (2005). A modular concept of phenotypic plasticity in plants. New Phytologist, 166(1), 73-82. doi:10.1111/ j.1469-8137.2004.01310.x. [ Links ]
Di Rienzo, J. A., Macchiavelli, R., & Casanoves, F. (2009). Modelos mixtos en InfoStat. Recuperado de https://academic.uprm.edu/rmacchia/agro6998/TutorialMMesp.pdf [ Links ]
Dias-Filho, M. B. (2002). Photosynthetic light response of the C4 grasses Brachiaria brizantha and B. humidicola under shade. Scientia Agricola, 59(1), 65-68. doi:10.1590/ S0103-90162002000100009. [ Links ]
Dohn, J., Dembélé, F., Karembé, M., Moustakas, A., Amévor, K. A., & Hanan, N. P. (2013). Tree effects on grass growth in savannas: Competition, facilitation and the stressgradient hypothesis. Journal of Ecology, 101(1), 202-209. doi:10.1111/1365-2745.12010. [ Links ]
Durr, P. A., & Rangel, J. (2003). The response of Panicum maximum to a simulated subcanopy environment: 2. soil x shade x water interaction. Tropical Grasslands, 37, 1-10. Recuperado de https://www.tropicalgrasslands.asn.au/TropicalGrasslandsJournalarchive/Abstracts/Vol_37_2003/Abs_01_03_pp01_10.html. [ Links ]
Edwards, E. J., & Smith, S. A. (2010). Phylogenetic Analyses Reveal the Shady History of C₄ Grasses. Proceedings of the National Academy of Sciences of the United States of America, 107(6), 2532-2537. doi:10.1073/pnas.0909672. [ Links ]
Giraldo-Cañas, D. (2010). Distribution and invasion of C 3 and C 4 grasses (Poaceae) along an altitudinal gradient in the Andes of Colombia. Caldasia, 32(1), 65-86. Recuperado de https://www.unal.edu.co/icn/publicaciones/caldasia.htm [ Links ]
Goméz, M., Navarro, O., & Pérez, A. (2016). Evaluacion de la frecuencias de corte del pasto guinea mombaza (Megathyrsus maximus, Jacq ), en condiciones de sol y sombra natural en el municipio de Sampués, Sucre-Colombia. Revista Colombiana de Ciencia Animal, 8, 283-292. [ Links ]
Guenni, O., Seiter, S., & Figueroa, R. (2008). Growth responses of three Brachiaria species to light intensity. Tropical Grasslands, 42(1), 75-87. [ Links ]
Guerin, G. R., Wen, H., & Lowe, A. J. (2012). Leaf morphology shift linked to climate change. Biology Letters, 8(5), 882 886. doi:10.1098/rsbl.2012.0458. [ Links ]
Holdridge, L. R. (1971). Forest environments in tropical life zones; a pilot study. Recuperado de https://www.searchworks.stanford.edu/view/609670 [ Links ]
Martínez, J., Cajas, Y. S., León, J. D., & Osorio, N. W. (2014). Silvopastoral systems enhance soil quality in grasslands of Colombia. Applied and Environmental Soil Science, 2014, Article ID 359736. doi:10.1155/2014/359736. [ Links ]
Medinilla-Salinas, L., Vargas-Mendoza, M. D. la C., López-Ortiz, S., Ávila-Reséndiz, C., Campbell, W. B., & Gutiérrez-Castorena, M. del C. (2013). Growth, productivity and quality of Megathyrsus maximus under cover from Gliricidia sepium. Agroforestry Systems, 87(4), 891-99. doi:10.1007/s10457-013-9605-1. [ Links ]
Obispo, N. E., Espinoza, Y., Gil, J. L., Ovalles, F., & Rodríguez, M. F. (2008). Efecto del sombreado sobre la producción y calidad del pasto guinea (Panicum maximun) en un sistema silvopastoril. Zootecnia Tropical, 26(3), 285-288. [ Links ]
Olivero-Lora, S. (2011). Functional trait approach to assess the ecological processes of drought tolerance and water use efficiency in silvopastoral systems in Rivas Department, Nicaragua. Turrialba, Costa Rica: Centro Agronómico Tropical de Investigación y Enseñanza (CATIE). [ Links ]
Ørskov, E. R., Hovell, D., & Mould, F. (1980). The use of the nylon bag technique for the evaluation of feedstuffs. Tropical Animal Production, 5(3), 195-203. Recuperado de https://www.fao.org/Ag/aga/AGAP/FRG/TAP53/53_1.pdf [ Links ]
Paciullo, D. S. C., Gomide, C. A. M., Castro, C. R. T., Maurício, R. M., Fernandes, P. B., & Morenz, M. J. F. (2017). Morphogenesis, biomass and nutritive value of Panicum maximum under different shade levels and fertilizer nitrogen rates. Grass and Forage Science, 72(3), 590-600. doi:10.1111/gfs.12264. [ Links ]
Pentón, G., & Blanco, F. (1997). Influencia de la sombra de los arboles en la composicion quimica y el rendimiento de los pastos. Pastos y Forrajes, 20(2), 101-110. Recuperado de https://www.payfo.ihatuey.cu/index.php?journal=pasto&page=article&op=view&path%5B%5D=1301&path%5B%5D=803 [ Links ]
Pezo, D., & Ibrahim, M. (1998). Sistemas silvopastoriles. Turrialba, Costra Rica: Centro Agronómico Tropical de Investigación y Enseñanza (CATIE). [ Links ]
Pinheiro, J., Bates, D., DebRoy, S., & Sarkar, D. (2018). nlme: Linear and Nonlinear Mixed Effects Models. Recuperado de https://www.cran.r-project.org/package=nlme. [ Links ]
R Core Team. (2018). The R Project for Statistical Computing. Recuperado de https://www.R-project.org/. [ Links ]
Rusch, G. M., Zapata, P. C., Casanoves, F., Casals, P., Ibrahim, M., & DeClerck, F. (2014). Determinants of grassland primary production in seasonally-dry silvopastoral systems in Central America. Agroforestry Systems, 88(3), 517-526. doi:10.1007/s10457-014-9711-8. [ Links ]
Sage, R. F., & McKown, A. D. (2006). Is C4 photosynthesis less phenotypically plastic than C3 photosynthesis? Journal of Experimental Botany, 57(2), 303-317. doi:10.1093/ jxb/erj040. [ Links ]
Santiago-Hernández, F., López-Ortiz, S., Ávila-Reséndiz, C., Jarillo-Rodríguez, J., Pérez-Hernández, P., & de Dios Guerrero-Rodríguez, J. (2016). Physiological and production responses of four grasses from the genera Urochloa and Megathyrsus to shade from Melia azedarach L. Agroforestry Systems, 90(2), 339-349. doi:10.1007/s10457-015-9858-y. [ Links ]
Silveira, O., Dos Santos, A. C., Rodrigues, M. O. D., Rodrigues, M. O. D., & Alencar, N. M. (2017). Productive efficiency of mombasa grass in silvopastoral system under pasture deferment and nitrogen fertilizer. Semina: Ciencias Agrarias, 38(5), 3307-3318. doi:10.5433/1679-0359. 2017v38n5p3307. [ Links ]
Sinclair, F. L. (1999). A general classification of agroforestry practice. Agroforestry Systems, 46(2), 161-180. doi:10.1023 /A:1006278928088. [ Links ]
Valadares, F., & Niinemets, Ü. (2008). Shade Tolerance, a Key Plant Feature of Complex Nature and Consequences. Annual Review Of Ecology, Evolution, and Systematics, 39, 237-257.doi:10.1146/annurev.ecolsys.39.110707. 173506. [ Links ]
Viafara, B., Clavero, T., & Araujo-Febres, O. (1997). Efecto del sombreamiento de samán (Pithecelobium saman Jacq. (Benth)) sobre el crecimiento y desarrollo del pasto guinea (Panicum maximum Jacq). Archivos Latinoamericanos de Producción Animal, 5(Supl. 1), 39-41. [ Links ]
Villanueva, C., Ibrahim, M., & Ríos, J. (2008). Disponibilidad de Brachiaria brizantha en potreros con diferentes niveles de cobertura arbórea en el trópico subhumedo de Costa Rica. Zootecnia Tropical, 26(3), 293-296. Recuperado de https://www.tspace.library.utoronto.ca/bitstream/1807/64242/1/zt08049.pdf [ Links ]
Wong, C. C., & Wilson, J. R. (1980). Effects of shading on the growth and nitrogen content of green panic and Siratro in pure and mixed swards defoliated at two frequencies. Australian Journal of Agricultural Research, 31(2), 269-285. doi:10.1071/AR9800269. [ Links ]
Acknowledgments
The authors wish to thank AGROSAVIA (formerly Corpoica) for the logistical and financial support in the development of this study. The authors also want to thank Ministerio de Agricultura y Desarrollo Rural de Colombia (madr) for financing the project in which the present research was carried out.
Received: May 07, 2018; Accepted: January 31, 2019