INTRODUCTION
Strawberry (Fragaria × ananassa) is a crop with high economic, nutritional, medicinal and culinary value (Lantz et al. 2010; PTP et al. 2013), to the point that it is considered a nutraceutical product, because of its high content of flavonoids, anthocyanins, phenolic compounds, and vitamins (Cao et al. 2011). Between 2015 and 2020, the area planted in strawberry crops in Colombia increased by 59 %, going from 1,656 hectares to 2,638, with an increase in production from 55,719 tons to 86,534, respectively (Minagricultura, 2021).
However, its production has a negative environmental impact because it depends on frequent applications of chemical fertilizers and products such as insecticides, fungicides, and herbicides, since it is highly susceptible to pathogens (Pritts, 2002; Vestberg et al. 2004). Edaphic pathogens such as Phytophthora, Pythium, Rhizoctonia, and Fusarium attack the crop. Other fungal diseases such as soft rot (Rhizopus stolonifer) and black rot (Mucor spp., Aspergillus niger, and Pythium spp.) are also common in postharvest. Anthracnose is also one of the major diseases caused by several species of the genus Colletotrichum, including C. acutatum, C. fragariae, and C. gloeosporioides (Chalfoun et al. 2011). These diseases have great importance because they can affect all plant tissues, such as stolons, crowns, stems, leaves, flowers, and fruits (Freeman & Katan, 1997), severely affecting productivity and profitability. Similarly, grey mold, caused by Botrytis cinerea, is one of the most destructive diseases during crop development and postharvest, causing severe economic losses, estimated at around 30 % of total production (Zhang et al. 2007). Even in postharvest, this pathogen is even more aggressive, affecting 95 % of the fruits 48 hours after harvesting (Li et al. 2019).
Traditionally, diseases caused by fungi are controlled with synthetic fungicides. However, there is increasing concern over the possible harmful effects these fungicides may have on the environment and human health. For the specific case of grey mold control, farmers use, among other strategies, chemical control with the application of the following active ingredients: Captan, Chlorothalonil, Iprodione, Procymidone, Fenhexamid, Diclofluanid, Tebuconazole, Methyl thiophanate, and Pyrimethanil (Fillinger et al. 2008, Leroux et al. 2010; FRAC, 2013). However, B. cinerea has a high capacity to become resistant to fungicides.
According to the above, the search for biological alternatives, such as the use of antagonistic microorganisms and/or biological control agents (BCA), is relevant for sustainable agriculture systems in order to achieve safe products (Guédez et al. 2009). Various studies have proven the individual effectiveness of some microorganisms used as biological control agents, such as fungi of the genus Trichoderma, arbuscular mycorrhizal fungi (AMF) and plant growth promoting bacteria (PGPB´s) antagonistic bacteria. However, research using functional mixtures of microorganisms to regulate pathogen populations and seek positive effects on crop yield and quality under field conditions have not been sufficient, due to the very complexity of soil-plant-microorganism-ambient interactions.
For the above the purpose of this research was to evaluate the effect of microbial consortia on the incidence of grey mold in strawberry under field conditions.
MATERIALS AND METHODS
Area location and time of the study. The experiment took place on the campus of the Universidad de Ciencias Aplicadas y Ambientales, U.D.C.A, at the research and teaching center “El Remanso”. The center is located at an altitude of 2560 m a.s.l, with geographic coordinates of N 4º35 W 74º04. Using a freely exposed production system, with 8 raised beds, 0.6 m width per bed, 0.4 meters between furrows, and 41.22 m long, plants were planted with three 0.3 m arrangements.
Agroclimatic data. Data reported by the Instituto de Hidrología, Meteorología y Estudios Ambientales (IDEAM) at the time that the experiment was developed, showed an average temperature of 14.1 °C, and precipitation of 506.5 mm; however, the high level of rainfall was during the experiment, reporting more than 50 % of the precipitation for that year. This increased the relative humidity, which registered values between 80 and 90 %.
Vegetable material and field distribution. In total, 1920 strawberry plants Monterey variety were used, distributed in eight blocks, and each block had eight treatments with 30 plants per treatment (Table 1).
Cultivation management. The agronomic management of the crop was focused on a clean production system, which completely restricted the use of chemicals for phytosanitary protection, instead, the cultural practices included phytosanitary pruning, with proper management of the remains of the infected plants, which were removed from the crop immediately and transferred to covered compost to decrease the inoculum of the infection and the dispersion of the pathogens. In addition, microbial inoculants were added at the time of transplantation, and re-inoculation was done three months after planting as a strategy for the regulation of the pathogens.
The cultivation system contemplated the elevation of beds or furrows, which were covered with mulch/plastic to reduce the presence of pathogens and weeds as is done in commercial crops on the savanna of Bogota. The irrigation system that was implemented used dripping, which was also used for fertilization. The first fertilization was done three months after sowing and for the study area, according to the soil analysis, with a recommended application of 533 g of Nitrogen (N), 666 g of Phosphorus (P) and 930 g of Potassium (K).
However, the fertilization was reduced to 50 % of the recommended dose because of the inoculation of biological products that are sensitive to optimum levels of fertilizers. In addition, the inclusion of biofertilization practice (the inoculums used as biological control agents, are also known as biofertilizers) decreases the demand for synthetic fertilizers by optimizing soil nutrient mineralization and plant availability (Li et al. 2017; Mondal et al. 2017). Finally, 250 g of N, 300 g of P and 400 g of K, applications were prepared in a mixture of 11 L of water and applied for five minutes once a week for four weeks. The subsequent fertilization was done six months to the ninth month (after sowing) increasing the dose of calcium nitrate to 300 g (N), 380 g of diammonium phosphate (P) and 480 g of potassium nitrate (K), once a week for 4 weeks.
Experimental inoculum. Microbial inoculants and their bioregulatory effect on pathogens are listed in table 2. The selection of microbial inoculants and consortia and the mixture of these were carried out to potentiate the beneficial effects for plant growth and protection against B. cinerea, knowing the multifunctionality as plant growth biostimulants and control agents biological of each of the microorganisms that made up the mixtures. However, the mixture was also made to observe the compatibility and/or functional complementarity of these microorganisms. Each plant per treatment was inoculated with 100 mL of solution plus an adjuvant (Agrotin®) 2 cm3L-1 at the time of transplantation and three months after the establishment of the crop.
Presence or absence of grey mold. The incidence of grey mold disease was evaluated in all plants, 90 days after transplantation. Only the incidence of the disease in fruits, as these are the main organ of interest in the production, was taken into account for the collection of the information, and for marking the plants according to diagnosis (diseased). The presence of at least one fruit affected by the disease was considered a diseased plant.
Botrytis cinerea natural inoculum. B. cinerea was not introduced to the crop, but was present in the soil as sclerotia, due to the previous sowing of strawberry Albión variety, during the semester immediately before the experiment, which presented estimated losses of over 30 % due to the presence of grey mold disease.
To monitor the grey mold disease at the time of sampling, the presence of the disease in fruits. After the field count, samples were taken to the vegetable sanitary laboratory in the Universidad de Ciencias Aplicadas y Ambientales U.D.C.A, to perform the microscopic observation of the pathogen and corroborate the presence of the disease in affected fruits.
Experimental design and associated variables. A simple factorial design was used in a randomized complete block design with eight blocks and eight treatments and 30 plants per treatment. The variable response was associated with the presence-absence dichotomy of grey mold and the explanatory variables involved blocking factors and biological treatments. The variables associated with the diagnosis of the disease (presence-absence) were evaluated in addition to the proportion of dead plants that were excluded from the modeling process since some of them died from causes different from the disease; for example, those that died from stress after transplantation. Table 1 shows the treatments and distribution of the treatments. The diagnosis was disaggregated by blocks and treatments.
Statistical analysis. Methodology for data analysis: The explanatory variables in logistic regression can be quantitative or qualitative, that is, factors such as treatments or blocks and covariates associated with physical and chemical properties of soil, air, and water. One of its advantages is that the interpretation of the model is possible by probabilities or disparity coefficients, which are a function of the model parameter (Stokes et al. 2012).
The statistical analysis included the descriptive component as the inferential. In the first case, the diagram of the spatial distribution of the plants was constructed according to the diagnosis of the disease, discriminated by treatments and blocks. Subsequently, the crossed tables were obtained for the proportion of plants according to diagnosis and the corresponding block, accompanying each proportion with the respective marginal count. A bar chart was then presented for diagnosis only in the levels of presence and absence of the disease by treatments and blocks. In the inferential component, a logistic regression model for the response associated with the diagnosis was adjusted using treatments and blocking as explanatory variables. Finally, the table of proportions predicted or adjusted by the model was constructed for each explanatory variable, values being compared with the observed values were sufficient reason to validate the model. The diagram for visualization of the spatial pattern as well as the adjustment of the logistic regression model was made using free software R. The likelihood ratio test was used to verify the fit of the model, comparing the complete model with the reduced model (only treatments effect).
RESULTS AND DISCUSSION
The presence of grey mold disease in strawberry fruits was evident, fruits collected in the field were taken to the plant health laboratory of the U.D.C.A, to make the respective macro and microscopic observations (Figure 1).
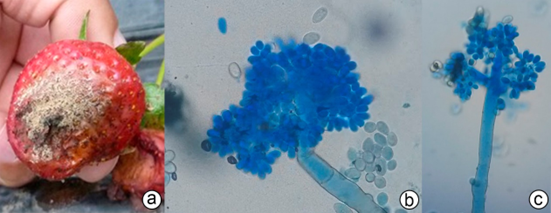
Figure 1 Presence of grey mold disease in the experimental field. a) Strawberry fruit with symptoms of grey mold; c) and d) microscopic observation of Botrytis cinerea conidiophores (40 and 10X).
The spatial representation of the disease diagnosis in healthy (green) and diseased (red) levels is seen in figure 2. The blank spaces between furrows and plants of each line correspond to dead plants (which were discarded from all analyzes once detected). The figure 2 fits the actual scheme of the sowing method and the coordinates (x, y) represents the measurements in centimeters.
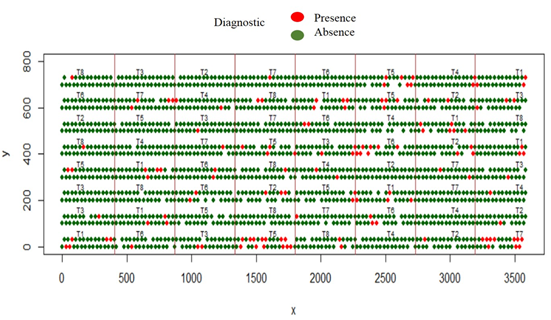
Figure 2 Spatial distribution of the disease diagnosis (Grey mold), according to treatment and shaped blocks 90 days after sowing.
For the inferential component, a logistic regression model was fitted using treatments and blocks as a factor and, as a response, the presence or absence of grey mold in fruits. The binary response variable (denoted by y), associated with the presence or absence of grey mold was written as a function of two predictor variables (denoted by x and z), namely the type of treatment (x) and the block (z).
Results showed statistical significance for the intercept and the coefficient associated with the treatments (p <5 %), not for the effect of the blocks (Table 3). Equation 1 was written as:
Therefore, the incidence of the grey mold disease estimated for all blocks and treatments is obtained by substituting the values of i and j associated with the treatments and blocks, respectively. The estimated values by the model next to a visual diagram (Figure 3), were used to rank the treatments according to the response.
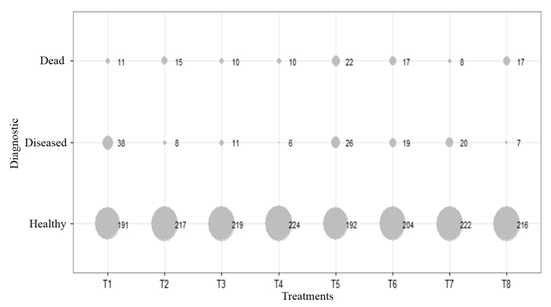
Figure 3 Marginal distribution of plant counts according to diagnosis (healthy, diseased, dead) for treatments: T1 Control (C), T2 Trichoderma (T), T3 Bacteria (B), T4 Mycorrhizal fungi (M), T5 (T + B), T6 (T + M), T7 (B + M) and T8 (T + B + M).
In addition to the spatial distribution, the estimation of statistical significance coefficients and distribution of prevalence according to diagnosis (in percentage), healthy, diseased and dead plants frequency per treatment was obtained (Figure 3). The values of the model adjustment (proof of the likelihood ratio) yielded a significance value of less than 5 %, placing the reduced model (without block effect) as a better model, as compared to the complete model. This result was consistent with the value of the null deviation and the residual deviation of the model.
The size of the circles is proportional to the frequency of the data, so the higher count is associated with healthy plants in both treatments and blocks, and the smaller points are associated with the low presence of grey mold disease. The treated plants were compared to the control in the line of diseased plants.
In figure 3, it is observed the low presence of grey mold disease, noting the graphic variability in the small size of the circles for all the biological treatments applied, with percentages of incidence of the disease in ascending order of: 2.6 (T4); 3.1 (T8); 3.6 (T2); 8.5 (T6); 8.6 (T7); 11.9 (T5), compared with control plants, which were not inoculated, nor treated with any chemical and had the highest percentage of incidence of the disease 16.6 (T1). Similar results were found in grapes, where B. cinerea had effective control with using different biological control agents (Pertot et al. 2017).
It was observed that the lowest incidence rates of grey mold disease are possibly related to the presence of mycorrhizal fungal consortium (Figure 3). These results may suggest that mycorrhizal fungi are strong competitors for space and nutrients in the rhizosphere (Brimner & Boland, 2003; Selosse et al. 2004; Pozo & Azcón-Aguilar, 2007; Avis et al. 2008; Wehner et al. 2010), are capable of rapidly colonizing the interior of the roots and extending a network of hyphae to the exterior, thus hindering the passage and mobility of pathogens, and also stimulate the plant defense system (Hause et al. 2007; Hause & Schaarschmid, 2009). Indirectly, hyphae produce exudates, which can modify the microbiome, and this modulates interactions with other organisms present in the rhizospheric zone (Finlay, 2004).
Regarding the microbial consortium composed of all microorganisms used in this research (T8), the incidence of B. cinerea was low at 3.1 %, placing it as the second-best treatment, compared to the control and the other treatments. This result may suggest the existence of functional compatibility of the consortium, in terms of the biological regulation of pathogens. In addition, a certain number of soil microorganisms stimulate the predetermined, and internal protection and defense system of plants, diminishing the biotic or abiotic stress (Kapoor et al. 2008).
Even though the results of this research seem so strong, it is difficult to predict the result of the interactions between beneficial plants and soil microorganisms, even more among the species of microorganisms used (Vázquez et al. 2000). However, the interactions between microorganisms are complex and synergic effects can be presented that potentiate the benefits for the plant or, on the contrary, antagonistic effects or simply that no effect occurs (Cano, 2011).
According to Bisutti et al. (2017), the biological control is complex and even more so with microbial mixtures, elucidating the mode of action is difficult, but microorganisms directly exert a beneficial effect in the promotion of plant growth and the strengthening of plants, against pathogens and adverse environmental conditions.
The use of biological control agents for managing diseases represents a viable alternative to replace or reduce applications of chemical fungicides (Cotes, 2014). In this investigation, the microbial consortia possibly acted jointly, to a different extent, but with positive results regarding the reduced incidence of grey mold disease, for all biological treatments, compared with plants without inoculation. This result may be due to what was suggested by Pertot et al. (2017), who points out that the combined strategy of different microbial biological control agents did not differ from the results when the individual treatments were applied, for the control of B. cinerea in grapes, which indicates that the microorganisms do not interfere negatively between them, and they could occupy different ecological niches. Likewise, similar results were presented by Pertot et al. (2008), using a different microbial consortium, composed of Ampelomyces quisqualis T. harzianum T39 and Bacillus subtilis, but to a lesser extent than chemical fungicides; however, biological control agents, reduce the use of chemical fungicides and can be applied alternately as part of an integrated disease management program.
According to the results of this research, the treatment T2 (T. harzianum), showed to be the third best treatment, very similar to the treatments T4 and T8 and compared with the control plants. Similar results were reported by Merchán-Gaitán et al. (2014), finding that in strawberry plants, the control obtained a greater incidence value of B. cinerea, than the treatments in which the biocontrollers (T. harzianum and T. lignorumpor) were applied (Ventana and Camino Real varieties).
T. harzianum, is one of the most widely distributed biological control agents in the world with a broad spectrum of pathogens that it regulates. It is also an excellent competitor, with high growth rates that surpasses most pathogens in vitro and is characterized by having different mechanisms and modes of action for bioregulation such as competition, mycoparasitism, production of lytic enzymes, antibiosis, production of siderophores, and stimulation of plants defense system (Harman et al. 2004; Harman, 2006). The results of this research concur with the literature because T. harzianum has a wide antagonistic potential that reduced the incidence of B. cinerea, compared with the plants that were not inoculated.
In this regard, Yang et al. (2009), in laboratory conditions, tested the bioregulatory efficiency of T. harzianum on B. cinerea isolated from the strawberry crop, obtaining positive results and mentioned that T. harzianum possesses a constitutive elicitor protein that acts against B. cinerea and other pathogens such as Rhizoctonia solani and Fusarium oxysporum. In the same way, Guédez et al. (2009), under similar conditions, dual tests in the laboratory, observed that the antagonist T. harzianum is a good competitor (rapid growth), and possibly its main mechanism of action is mycoparasitism, in common strawberry postharvest pathogens, such as Rhyzopus stolonifer, Mucor spp., Penicillium digitatum, Rhizoctonia solani, Aspergillus niger and Pythium spp.
In contrast to the above, the treatments T5, T6, and T7, even though they had lower percentages of incidence of the disease 11.9, 8.5 and 8.6 %, respectively, compared to the control (T1) 16.6 %, the response was not as noticeable as the other treatments analyzed. It is possible that, as previously mentioned, these mixtures of microorganisms did not present adequate complementation in the regulation of the disease; some of the different microbial consortia members could alter the bioregulatory effect of others, affecting the effectiveness or efficiency of the consortium in the pathogen biological regulation.
Results of this investigation showed that the inoculation and co-inoculation of mycorrhizal fungi, antagonistic bacteria, and T. harzianum, interact positively in favor of the plants’ health, managing to reduce the incidence of B. cinerea in strawberry fruits.