INTRODUCTION
Quinoa is a transient crop currently produced in several areas of the world, it is characterized by being adaptable to different edaphoclimatic conditions, resulting in region-specific quinoa cultivars (Bazile et al., 2016). These aspects bring about the concept "ecophysiology" defined as the discipline that explains the relationship between the physiological behavior of plants and the external conditions that affect their performance (Larcher, 2003), whereby aspects such as practices and interactions with their physical and biological environments contribute to the distinctive characteristics of quinoa grains grown in specific regions (García-Parra et al., 2020a).
In this sense, weather and soil characteristics, associated with the production area, have been studied in the last few years. Bosque-Sanchez et al. (2003) were the first scientists to talk about ecophysiology in quinoa. More recently, Murphy and Matanguihan (2015) considered the importance of seed characteristics of different quinoa cultivars, which was similar to that proposed by Reguera et al. (2018). Ruiz et al. (2014) highlighted the existence of edaphic microorganisms, capable of carrying out symbiotic relationships with different quinoa cultivars, however, despite the importance of these associations, their relevance and analysis have been scarce studied.
Among the great diversity of edaphic organisms, arbuscular mycorrhizal fungi are those with the greatest capacity to carry out symbiosis with quinoa roots (USDA, 2016). In this sense, this type of symbiosis is the most beneficial interaction between edaphic microorganisms and roots (Begum et al., 2019; Teste et al., 2020). The phylum Glomeromycota is the most important group of fungi in this activity, and therefore, it has been recognized that about 80% of terrestrial plant species including quinoa, manage to form a symbiosis with arbuscular mycorrhizae (Trouvelot et al., 2015), even though for many years, it was reported that some species belonging to the Chenopodiaceae and now Amaranthaceae family, it did not carry out edaphic symbiosis with fungi (Rydlová and Vosfitka, 2001; Chaudhry et al., 2005).
The mutualistic interaction between the mycorrhizal fungus and plant roots is based on the exchange of nutrients between these two actors, where the plant supplies carbon, while the fungus favors the activity of nutrient and water absorption, which takes place at the moment when some structures of the fungus colonize the cortical cells of the plant roots consolidating complex frameworks called arbuscules (Vierheilig, 2004; Janouskova et al., 2017). According to the above information, it becomes evident that this direct link between soil and quinoa allows to carry out a greater approach between the ecophysiological behavior of quinoa with its environment, which is important for the productive and scientific community of this species.
However, the high diversity of both fungi and cultivars of quinoa, as well as the edaphoclimatic conditions where the crop is established, make their relationship dynamic, increasing the interest of producers and research centers to know the characteristics and benefits of arbuscular mycorrhizae. For this reason, this work aimed to present a systematic review of the main aspects related to mycorrhizal activity between fungi and quinoa plants.
MATERIALS AND METHODS
A systematic review was carried out based on the PRISMA methodology (Preferred Reporting Items for Systematic Reviews and Meta-Analysis) (Urrútia and Bonfill, 2010), with the research question: what is the relationship described by the scientific literature between fungi with mycorrhizal capacity and quinoa plants?
Search and data collection
To ensure the success and sensitivity of the search, the descriptor "soil", "quinoa" was consolidated using the Boolean operator AND in the conjugation "soil AND quinoa". The exploration included all documents that find the search path in the title, abstract, and keywords initially; contemplating articles, books, book chapters, and conferences published in the Scopus and Clarivate Web of Science (WoS) databases following the methodology proposed by Yepes et al. (2018).
Selection criteria
Within the scientific papers obtained, a second filter was developed that based its selection on the following criteria: papers from journals were indexed in the Scimago Journal & Country Rank SJR database; papers published between 2000 and 2021 and that described theoretical aspects and scientific experiences of the activity of edaphic fungi with quinoa. In addition, special importance was given to documents that included (i) diversity of edaphic fungi with mycorrhizal capacity in soils suitable for quinoa cultivation; (ii) importance of fungi that favor the nutrition of this species, and finally (iii) importance on the biological response of the species. All documents related to quinoa and that did not contemplate explanation, use or extermination with arbuscular mycorrhizal fungi were excluded.
Data processing and analysis
To determine the dynamics of the development of research related to soil AND quinoa, all the documents obtained under this search formula in the two databases were analyzed, taking the results from each of them and eliminating duplicates with the help of Mendeley reference manager. Subsequently, the documents found were organized in Excel® software by year, by the number of publications and the total. In addition, the main countries and research areas were detailed. Additionally, an analysis of publications was performed through non-linear regressions to determine their global trend, modeling the information in Sigmoidal 3-4-5 parameters, Logistic 3-4, Weibull 4-5 Gompertz 3, Hill 3-4 and Chapman 3-4 through the statistical program Sigmaplot (SystatSoftware Inc., San Jose USA). Data fit was selected through the coefficient of determination (R2) and significance of the data (P<0.05) following the methodology used by García-Parra et al. (2020b).
RESULTS AND DISCUSSION
Through the analysis, it was determined that there are 239 documents published in the selected databases, distributed in research articles (205), review articles (11), book chapters (10), conferences (9), notes (2) and short review articles (1) between 2000 and 2020. In this context, the trend of research exposing the characteristics, use and management of soil, as well as its physical, chemical and microbiological properties with incidence on the biological performance of quinoa has grown exponentially, which is evidenced by the inflection point (2029) of the best fit model, which for this case was sigmoidal 3 parameters (R2 0.997; P<0.05) (Table 1).
Table 1 Nonlinear regression models meeting the parameters for the search path "soil AND quinoa" analyzed since 2000.
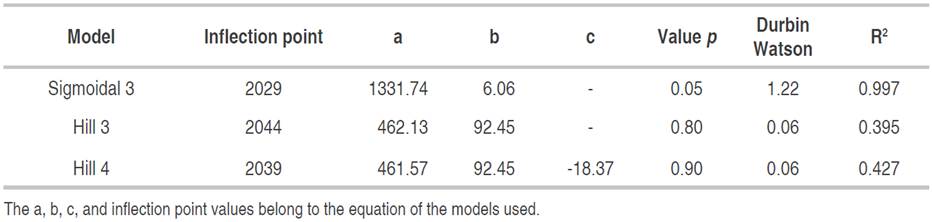
According to what was proposed by Escobar and Zartha (2017), the search areas of this systematic research are the key to incoming phase (Figure 1), which manifests an opportunity to produce this species, given as a consequence of the novel discovery of the symbiotic association between fungi and quinoa. In this sense, the search conjugation identified nine publications that met the criteria of the PRISMA methodology and that open in a specific way the relationship between quinoa plants and mycorrhizal activity, highlighting that quinoa has been recognized since 2013 as a promising crop against the effects of climate change, which is the reason why identifying the symbiotic association of this species with mycorrhizal fungi could enhance its production under more difficult edaphoclimatic conditions.
The regions with the greatest publication focus on quinoa and its relationship with the ecophysiological environment are developed countries, while developing countries such as those belonging to Latin Americana (Table 2) present contributions in a smaller proportion (García-Parra and Plazas-Leguizamón, 2019). In the case of Colombia, it has a participation of 0.83% in the world scientific production. In the case of Denmark, Chile, and Peru, the recognition in the study of quinoa is framed in the obtaining of the Plant Variety Certification (COV), whose advantage lies in the genetic improvement activity and its in-depth study without having to refer to the country of origin (Bazile et al., 2014).
Table 2 Countries with the highest number of publications on the relationship between soil and quinoa (239 publications according to the Scopus database).
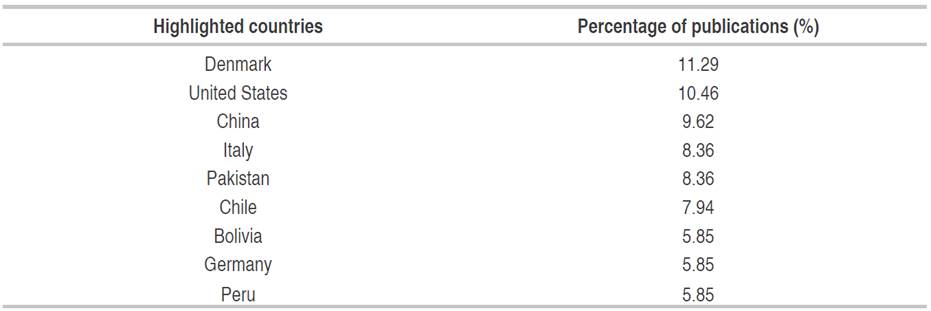
Given the interdisciplinarity involved in the production of quinoa crops, the development of publications addresses main areas such as agriculture and biological sciences (79.91%), environmental sciences (18.41%), biochemistry, genetics, and molecular biology (12.97%), engineering (5.85%), social sciences (5.02%) and earth and planetary sciences (4.6%), immunology and microbiology (4.6%), medicine (3.76), chemistry (2.51%), energy (2.51%) among others (11.29). The above demonstrates the growing interest that continues to develop the crop in aspects such as interactive dynamics with the soil, mainly, focused on the strategies used by the plant to capture water and nutrients (Choukr-Allah et al., 2016).
It is relevant to highlight the nine publications that were most relevant according to the search for "soil AND quinoa" which contributed to the development of the discussion of the relationship between mycorrhizal fungi and their symbiosis with quinoa plants (Table 3).
Consequently, the investigations listed in the Table 3 present the most important research advances in the world, where aspects related to fungal diversity are highlighted, its importance in the nutrition of the species, the transport of elements from the soil to the plant interior, as well as metabolic costs in symbiotic activity.
Importance of mycorrhizal diversity
The diversity of edaphic microorganisms has been studied mainly during the last decades, however, its study has been difficult due to the microscopic dimension of many of these species and the fact that many of them are hidden. In this sense, several factors affect their existence and their activity with other organisms, aspects that Landinez-Torres et al. (2019) recognize as determinants of fungal diversity, soil stability, and nutrient cycling.
All the fungi capable of establishing an arbuscular mycorrhizal symbiosis are grouped in the Glomeromycota phylum (Pedone-Bonfim et al., 2018); however, fungal families differ in their colonization strategy to the root, as demonstrated in Glomerales that carry out mycorrhization through hyphal fragments, while Diversisporales do it by spores, which is reflected in the colonization speed and the rapid adaptability of the plant to a particular stress condition (Trouvelot et al., 2015). According to this, differences in growth strategies between these fungi imply that soil management can greatly impact the diversity of arbuscular mycorrhizal fungi. For example, in quinoa, it has been reported that the greatest mycorrhizal formation occurs above 3700 masl and its fungal colonization dynamic is very similar to that of Poaceae (Urcelay et al., 2011).
Although research related to fungal diversity in quinoa is scarce, Cai et al. (2020) have reported a high presence of phylum such as Ascomycota, Basidiomycota, Zygomycota and Chydiomycota in the rhizospheric zone of quinoa plants, changing their percentage of abundance in relation to the altitude where the samples were collected. However, the authors recognize a high percentage of unidentified fungi that manage to carry out mycorrhization with this plant. This is an activity that is not only carried out in quinoa but also in other cultivated plants of agronomic interest such as maize (Zea mays), wheat (Triticum aestivum), and soybean (Glycine max), which naturally present a high diversity of mycorrhization (Renaut et al., 2020).
As it was discussed above, colonization of plant roots by edaphic fungi is an activity that occurs in many plants, and thus arbuscular mycorrhizal fungi (AMF) is not specific for a particular plant species. For example, Ascomycota is recognized for being the largest group of fungi capable of favoring nutrient uptake and generating a greater defense to pest and disease attack, while Basidiomycota has been recognized for its high colonization effectiveness in forested areas, where the presence of lignocellulosic material is abundant (Landinez-Torres et al., 2019). For the case of Zygomycota, its maximum performance has been manifested in soils where mechanical management and the use of external inputs is minimal, a habit that is very similar to the Chytridiomycota phylum (Panelli et al., 2017).
Thus, the question of AMF being associated with quinoa plants has been addressed in a few studies. This situation generated that the identification of the diversity of fungi capable of performing mycorrhization with this species will be carried out with detection methods based on DNA extraction and its amplification through PCR in three areas with different altitudes as reported by Cai et al. (2020). However, research has also analyzed the amount of spores present in rhizospheric soil, given that a relationship is found with the percentage of root colonization (Isobe et al., 2007).
This shows the importance of developing research related to the edaphic microbiota of quinoa, focused on showing the diversity of fungi, not only from the phylum but also from the species, since this would facilitate the knowledge of the real mutualistic activity that is developed between these two organisms and thus identify any advantage that strengthens the resistance of quinoa to soils with a higher saline activity, extreme water stress or strong temperature changes.
Nutritional physiology and its relationship with AMF
The physiological activity of plants is strongly related to the surrounding climatic conditions and the physical, chemical, and biological characteristics of the soil (Taiz and Zeiger, 2006). This is the reason why achieving favorable conditions for quinoa cultivation determines the success of seed production and grain quality (Reguera et al., 2018; García-Parra et al., 2019). Thus, carrying out fertilization plans for the plant to generate stimuli that favor its yield is complex, as it depends largely on the quinoa cultivar to be used, soil characteristics, and even the type of production (foliage or grain). As a consequence of the multiple campaigns developed by different governmental and private entities regarding the adaptability of quinoa to areas where other crops cannot survive, most quinoa production systems are located in soils with low availability of nutrients, water, conditions of salinity and strong temperature changes; scenarios that in many cases generate changes at the physiological, phenological and morphological level of quinoa (Ruiz et al., 2014).
It is well known that a good AMF colonization occurs under conditions of low nutrient availability in plants or soil (Begum et al., 2019), where fungi colonize the root tissue of the plant and seek a greater exploration of soil area, to absorb nutrients and water, which are transported to the interior of the plant through the hyphal networks. However, this activity generates a physiological cost, which translates into a constant demand for carbon energy sources that facilitate the metabolic activity of the fungus; this interaction is estimated to demand between 10 and 30% of the photoassimilates produced by the plant, used for the formation, maintenance, and functionality of the mycorrhizal structure (Alarcón and Ferrera-Cerrato, 1999).
Nevertheless, all this biological activity is also determined by the root architecture of the plant (Bender et al., 2014). The root architecture of quinoa is recognized for being highly branched and with good development in the density of fine roots, which favors the ability to colonize the soil and increase the uptake of water and nutrients that are translocated by the plant and transported into biomass and seeds with greater efficiency in this species compared to others belonging to the Chenopodium subfamily (Alvarez-Flores et al., 2014). Therefore, once plants are colonized by AMF, nutrient uptake efficiency increases since nutrient uptake can occur through the root or the arbuscular mycorrhiza involving the hyphal structure of the fungus, this latter with a greater facility to explore areas where the root cannot reach (Liu et al., 2016).
Phosphorus
A morphological character of AMF in the plant is its penetration into the cortical cells of the root and the development of a prominent hyphal structure. However, this aspect is highly variable among plants, fungal groups, and edaphoclimatic characteristics (Kobae, 2019). In this sense, the theory that most accurately explains the interaction of these dynamics is the one that proposes that although AMF rapidly colonizes the interior of the root cell, the arbuscular structure does not completely fuse with the colonized cell, there is also no instantaneous flowing exchange, given that the fungal structure is surrounded by periarbuscular membranes that can change in thickness and composition according to the type of fungus, determining the rate of exchange of substances by the two organisms (Kobae and Hata, 2010; Camarena-Gutierrez, 2012).
It is well known that phosphorus is a vital element in plant physiology since this element is a structural part of genetic chains, metabolic energy, and accessory structures of cell membranes mainly (Shen et al., 2011). Nonetheless, plants encounter different difficulties when taking it from the soil, mainly due to its low mobility, the effect of acid pH, soil colloidal dynamics, and microbial activity (Marschner, 2012).
Consequently, the increase of arbuscular structures in the root tissue favors the expression of genes that facilitate the capture of phosphates from the fungal structure to the plant tissue, through proteins located in the periarbuscular membrane (Harrison et al., 2002). However, the dynamics developed for the exchange of elements such as phosphorus between the plant and the fungus are variable. This is because active colonization presents an accelerated movement of phosphorus, mainly when it is scarce. Furthermore, senescent colonization occurs when the hyphal network stops growing and, therefore, it is still unknown whether phosphorus mobility continues between the plant tissue and the arbuscular branch (Kobae et al., 2016) (Figure 2).
In quinoa plants, the combined effect of the application of edaphic phosphorus and AMF (Glomus mosseae and Glomus tortuosum) on physiological parameters has been studied, resulting in a significant increase in vegetative growth, chlorophyll content, photosystem II photochemical efficiency (Fv/Fm) and photosystem II potential activity (Fv/Fo) during the initial phase of the trial, followed by a decrease in physiological vigor after the application of the phosphorus treatments compared to the treatment without AMF inoculation; It was also found that quinoa plants with AMF application showed better physiological parameters except for root diameter (Chunhua et al., 2017).
According to different scientific antecedents, it is possible to indicate that the benefits offered by AMF to the host plant, demand a carbon cost, which are normally attributed to carbohydrate and lipid compounds product of the photosynthetic activity of the plant and that serve the fungus to support the growth of different structures such as hyphae and spores mainly (Keymer et al., 2017), which maintains the mutualistic activity of the organisms through a molecular dialogue between the plant and AMF, including complex networks of perception and signal transduction of genes from both symbiotic partners (Panelli et al., 2017). For the case of plants, bioactive signals are mainly given by strigolactones and (iso) flavonoids, while AMF secretes lipochitooligosaccharide (LCO) and short-chain chitin oligomer (Nanjareddy et al., 2017). At present, few studies have reported the relationship of symbiotic activity between fungi and quinoa, so more efforts are needed to investigate the mechanism that develops between these two organisms, mainly in the activity with phosphorus uptake.
Nitrogen
As a consequence of the high demand for nitrogen by the quinoa crop, its capture becomes crucial for the development of metabolism related to protein structuring and in the development of photosynthetic activity in this species (García-Parra et al., 2019). Thus, nitrogen is considered an essential element, given that it determines the phenological cycle of quinoa and the protein potential of its seeds, which is significantly higher compared to cereals (Bascuñán-Godoy et al., 2018b) but is strongly influenced by the availability and absorption of nitrogen in relation to soil type and organic matter turnover and, therefore, this characteristic is highly changeable between edaphic microclimates.
Although nutritional interactions related to nitrogen in quinoa cultivation, have been widely studied (González et al., 2009; Bascuñán-Godoy et al., 2018a; Bascuñán-Godoy et al., 2018b), quinoa production systems do not optimize the supply of this element and, on the contrary, make excessive use of nitrogen fertilizers. Because of its importance, AMF has established a relevant relationship with plants, no greater than that developed around phosphorus. AMF can take up nitrogen in the form of NO3 -, NH4 + and as organic nitrogen, however, the fungus presents a strong preference for NH4 +, which is the most assimilable form and of lower energy expenditure by the plant after reducing NO3 - into NO2 - by enzymatic action and this transformed into NH4 + by the effect of nitrite reductase (Fonseca-López et al., 2020). AMF colonization in quinoa has been studied over time and has been compared with other food crops such as wheat (Triticum vulgare), barley (Hordeum vulgare) and chickpea (Cicer arietinum), showing a low percentage of colonization in relation to the content of hyphae, vesicles, and arbuscules as was reported by Wieme et al. (2020), which determines that further study of the response of this species to AMF colonization and its relationship with nutrient transport should be consolidated.
In addition to the application and uptake of inorganic nitrogen, it has been established that AMF absorbs substantial amounts of organic nitrogen (Trouvelot et al., 2015) and that by the nature of the soil and climatic conditions such as temperature, its uptake into the plants is favored. In fact, in organic quinoa production systems the incorporation of behaved animal excreta and harvest residues has been used to increase the presence of nitrogen in the soil, expressing favorable results (Buckland et al., 2018; García-Parra et al., 2019), in benefit of the speed of mineralization of the material and the activity of organisms such as AMF and bacteria mainly.
Carbon cost due to the effect of symbiosis
Carbon demand by AMF is a considerable aspect for the colonized plant, as the fungus can receive between 4 and 20% of carbon photosynthetically fixed by the plant (Soudzilovskaia et al., 2019). Quinoa, as a transient plant, depends on an accelerated mobility of C during its productive cycle, so an imbalance during its development, would affect productivity, given that the low amount of reserves that it manages to accumulate for long periods in the leaves. AMF growth and nutrient acquisition depend on plant reserves, therefore, the mobility of C reserves and plant development could be significantly affected, as reported by Kellogg et al. (2021), who evaluated different quinoa cultivars that were subjected to mycorrhization and showed an increase in dry biomass production, while there was negative growth in plant height compared to the control treatment; an aspect in which the authors emphasized the importance of not colonizing with AMF in this species, given the heterogeneity that quinoa shows when colonized with these organisms.
However, the biochemical activity that is developed around the symbiosis between AMF and quinoa is very uncertain, so research is needed to detail its effect in relation to their interaction, in terms of the great diversity of fungi that can colonize and the wide diversity of quinoa cultivars.
AMF and water stress
The biological state of the soil and the water use of the plants play a key role in quinoa physiological behavior and grain quality. Nevertheless, given the campaigns developed by different governmental and private entities, the adaptability of quinoa in marginal areas, with problems of salinity, water availability, and nutritional deficit, this species has had to generate different metabolic strategies that allow its normal development. In this sense, it has been shown that climate change affects the phenological development of quinoa (Jaikishun et al., 2019; García-Parra et al., 2020a) and despite this, quinoa generates strategies for its adaptability as demonstrated Reguera et al. (2018), who found a differentiated behavior of quinoa, under different agroecological zones of production. Thus, the agronomic and management strategies of quinoa crops play a determining role in supplying water and nutrients to plants and, therefore, it is known that AMF can absorb and transport water and nutrients into the plant tissue, thus, an increase in the extension of fungal mycelium can be decisive in dry seasons.
This situation, in which the fungus manages to extend its mycelium, favors soil structuring, since the development of complex and branched networks can bring soil particles together and improve their structure (Rillig and Mummey, 2006), which together with the secretion of mucilages, polysaccharides and extracellular compounds such as glomalin and hydrophobins can increase the hydrophobic organic matter that creates more stable aggregates in the face of changes in soil water status (Rashid et al., 2016) and that would favor joint activity with organisms such as bacteria, which act freely in quinoa, and that would favor the availability of more nutrients for this crop and the retention of water in soil colloidal matrices.
Nevertheless, despite the knowledge gap regarding the symbiotic interaction between quinoa plants and AMF, it is possible to intuit that quinoa plants do not fully recognize AMF as a beneficial agent and, therefore, synthesize secondary metabolites such as phytoalexins, which have been found in high contents in quinoa and are capable of generating control by the attack of pathogenic fungi and pests (Yactayo-Chang et al., 2020).
Experiences of AMF in quinoa
Through the growing study of quinoa during the last decades, research has allowed the establishment of some scientific experiences that highlight the relationship between AMF and quinoa. In summary, this section will highlight the most relevant trials that relate to the mutualistic interaction of the two organisms mentioned above. In this sense, the search analysis determined that the research related to this topic has been developed basically in the United States, China, Japan, Finland, and Bolivia, while the diversity of fungi found in association with this plant is scarce (Table 4).
Wieme et al. (2020) reported that the symbiotic association between quinoa and fungi developed through the formation of structures such as hyphae, vesicles, and arbuscules, although their presence is significantly lower compared to other crops of food interest such as wheat, chickpea, and barley. Additionally, Urcelay et al. (2011) identified abundant radical colonization of Olpidium, with an abundant presence of mycelium, vesicles, and arbuscules, however, this fungus is recognized for its pathogenic capacity, which draws the attention of researchers and suggests the importance of carrying out trials around the activity of these two organisms.
CONCLUSIONS
Quinoa presents a response to the presence and colonization of fungi with mycorrhizal capacity, which can manifest itself as a beneficial agent, capable of developing symbiotic activity, or generate physiological and biochemical responses that recognize this organism as a pathogenic agent. Because of the uncertainty expressed by quinoa in the presence of fungi with mycorrhizal capacity, the references highlight the need to study the behavior that different quinoa cultivars may show when applied under controlled and field conditions.
In general, it has been reported that the major fungal structure found inside the root cells of quinoa is mycelium, which does not necessarily indicate that there is a constant activity of exchange of substances that benefit both organisms.